https://en.wikipedia.org/wiki/History_of_Earth#/media/File:Geologic_Clock_with_events_and_periods.svg
Eon | Time (mya) | Description |
---|---|---|
Hadean | 4,540–4,000 | The Earth is formed out of debris around the solar protoplanetary disk. There is no life. Temperatures are extremely hot, with frequent volcanic activity and hellish-looking environments (hence the eon's name, which comes from Hades). The atmosphere is nebular. Possible early oceans or bodies of liquid water. The Moon is formed around this time probably due to a protoplanet's collision into Earth. |
Archean | 4,000–2,500 | Prokaryote life, the first form of life, emerges at the very beginning of this eon, in a process known as abiogenesis. The continents of Ur, Vaalbara and Kenorland may have existed around this time. The atmosphere is composed of volcanic and greenhouse gases. |
Proterozoic | 2,500–538.8 | The name of this eon means "early life". Eukaryotes, a more complex form of life, emerge, including some forms of multicellular organisms. Bacteria begin producing oxygen, shaping the third and current of Earth's atmospheres. Plants, later animals and possibly earlier forms of fungi form around this time. The early and late phases of this eon may have undergone "Snowball Earth" periods, in which all of the planet suffered below-zero temperatures. The early continents of Columbia, Rodinia and Pannotia, in that order, may have existed in this eon. |
Phanerozoic | 538.8–present | Complex life, including vertebrates, begin to dominate the Earth's ocean in a process known as the Cambrian explosion. Pangaea forms and later dissolves into Laurasia and Gondwana, which in turn dissolve into the current continents. Gradually, life expands to land and familiar forms of plants, animals and fungi begin appearing, including annelids, insects and reptiles, hence the eon's name, which means "visible life". Several mass extinctions occur, among which birds, the descendants of non-avian dinosaurs, and more recently mammals emerge. Modern animals—including humans—evolve at the most recent phases of this eon. |
Geologic time scale
The history of the Earth can be organized chronologically according to the geologic time scale, which is split into intervals based on stratigraphic analysis.[2][22] The following five timelines show the geologic time scale to scale. The first shows the entire time from the formation of the Earth to the present, but this gives little space for the most recent eon. The second timeline shows an expanded view of the most recent eon. In a similar way, the most recent era is expanded in the third timeline, the most recent period is expanded in the fourth timeline, and the most recent epoch is expanded in the fifth timeline.





Thousands of years (5th)
Solar System formation
The standard model for the formation of the Solar System (including the Earth) is the solar nebula hypothesis.[23] In this model, the Solar System formed from a large, rotating cloud of interstellar dust and gas called the solar nebula. It was composed of hydrogen and helium created shortly after the Big Bang 13.8 Ga (billion years ago) and heavier elements ejected by supernovae. About 4.5 Ga, the nebula began a contraction that may have been triggered by the shock wave from a nearby supernova.[24] A shock wave would have also made the nebula rotate. As the cloud began to accelerate, its angular momentum, gravity, and inertia flattened it into a protoplanetary disk perpendicular to its axis of rotation. Small perturbations due to collisions and the angular momentum of other large debris created the means by which kilometer-sized protoplanets began to form, orbiting the nebular center.[25]
The center of the nebula, not having much angular momentum, collapsed rapidly, the compression heating it until nuclear fusion of hydrogen into helium began. After more contraction, a T Tauri star ignited and evolved into the Sun. Meanwhile, in the outer part of the nebula gravity caused matter to condense around density perturbations and dust particles, and the rest of the protoplanetary disk began separating into rings. In a process known as runaway accretion, successively larger fragments of dust and debris clumped together to form planets.[25] Earth formed in this manner about 4.54 billion years ago (with an uncertainty of 1%)[26][27][4] and was largely completed within 10–20 million years.[28] The solar wind of the newly formed T Tauri star cleared out most of the material in the disk that had not already condensed into larger bodies. The same process is expected to produce accretion disks around virtually all newly forming stars in the universe, some of which yield planets.[29]
The proto-Earth grew by accretion until its interior was hot enough to melt the heavy, siderophile metals. Having higher densities than the silicates, these metals sank. This so-called iron catastrophe resulted in the separation of a primitive mantle and a (metallic) core only 10 million years after the Earth began to form, producing the layered structure of Earth and setting up the formation of Earth's magnetic field.[30] J.A. Jacobs [31] was the first to suggest that Earth's inner core—a solid center distinct from the liquid outer core—is freezing and growing out of the liquid outer core due to the gradual cooling of Earth's interior (about 100 degrees Celsius per billion years[32]).
−4500 — – — – −4000 — – — – −3500 — – — – −3000 — – — – −2500 — – — – −2000 — – — – −1500 — – — – −1000 — – — – −500 — – — – 0 — |
| |||||||||||||||||||||||||||||||||||||||||||||
Tectonics, paleogeography and climate
At the end of the Proterozoic, the supercontinent Pannotia had broken apart into the smaller continents Laurentia, Baltica, Siberia and Gondwana.[153] During periods when continents move apart, more oceanic crust is formed by volcanic activity. Because young volcanic crust is relatively hotter and less dense than old oceanic crust, the ocean floors rise during such periods. This causes the sea level to rise. Therefore, in the first half of the Paleozoic, large areas of the continents were below sea level.[citation needed]
Early Paleozoic climates were warmer than today, but the end of the Ordovician saw a short ice age during which glaciers covered the south pole, where the huge continent Gondwana was situated. Traces of glaciation from this period are only found on former Gondwana. During the Late Ordovician ice age, a few mass extinctions took place, in which many brachiopods, trilobites, Bryozoa and corals disappeared. These marine species could probably not contend with the decreasing temperature of the sea water.[154]
The continents Laurentia and Baltica collided between 450 and 400 Ma, during the Caledonian Orogeny, to form Laurussia (also known as Euramerica).[155] Traces of the mountain belt this collision caused can be found in Scandinavia, Scotland, and the northern Appalachians. In the Devonian period (416–359 Ma)[22] Gondwana and Siberia began to move towards Laurussia. The collision of Siberia with Laurussia caused the Uralian Orogeny, the collision of Gondwana with Laurussia is called the Variscan or Hercynian Orogeny in Europe or the Alleghenian Orogeny in North America. The latter phase took place during the Carboniferous period (359–299 Ma)[22] and resulted in the formation of the last supercontinent, Pangaea.[59]
By 180 Ma, Pangaea broke up into Laurasia and Gondwana.[citation needed]
Cambrian explosion
The rate of the evolution of life as recorded by fossils accelerated in the Cambrian period (542–488 Ma).[22] The sudden emergence of many new species, phyla, and forms in this period is called the Cambrian Explosion. The biological fomenting in the Cambrian Explosion was unprecedented before and since that time.[58]: 229 Whereas the Ediacaran life forms appear yet primitive and not easy to put in any modern group, at the end of the Cambrian most modern phyla were already present. The development of hard body parts such as shells, skeletons or exoskeletons in animals like molluscs, echinoderms, crinoids and arthropods (a well-known group of arthropods from the lower Paleozoic are the trilobites) made the preservation and fossilization of such life forms easier than those of their Proterozoic ancestors. For this reason, much more is known about life in and after the Cambrian than about that of older periods. Some of these Cambrian groups appear complex but are seemingly quite different from modern life; examples are Anomalocaris and Haikouichthys. More recently, however, these seem to have found a place in modern classification.[156]
During the Cambrian, the first vertebrate animals, among them the first fishes, had appeared.[123]: 357 A creature that could have been the ancestor of the fishes, or was probably closely related to it, was Pikaia. It had a primitive notochord, a structure that could have developed into a vertebral column later. The first fishes with jaws (Gnathostomata) appeared during the next geological period, the Ordovician. The colonisation of new niches resulted in massive body sizes. In this way, fishes with increasing sizes evolved during the early Paleozoic, such as the titanic placoderm Dunkleosteus, which could grow 7 meters (23 ft) long.[157]
The diversity of life forms did not increase greatly because of a series of mass extinctions that define widespread biostratigraphic units called biomeres.[158] After each extinction pulse, the continental shelf regions were repopulated by similar life forms that may have been evolving slowly elsewhere.[159] By the late Cambrian, the trilobites had reached their greatest diversity and dominated nearly all fossil assemblages.[160]: 34
Colonization of land
Oxygen accumulation from photosynthesis resulted in the formation of an ozone layer that absorbed much of the Sun's ultraviolet radiation, meaning unicellular organisms that reached land were less likely to die, and prokaryotes began to multiply and become better adapted to survival out of the water. Prokaryote lineages[161] had probably colonized the land as early as 2.6 Ga[162] even before the origin of the eukaryotes. For a long time, the land remained barren of multicellular organisms. The supercontinent Pannotia formed around 600 Ma and then broke apart a short 50 million years later.[163] Fish, the earliest vertebrates, evolved in the oceans around 530 Ma.[123]: 354 A major extinction event occurred near the end of the Cambrian period,[164] which ended 488 Ma.[165]
Several hundred million years ago, plants (probably resembling algae) and fungi started growing at the edges of the water, and then out of it.[166]: 138–140 The oldest fossils of land fungi and plants date to 480–460 Ma, though molecular evidence suggests the fungi may have colonized the land as early as 1000 Ma and the plants 700 Ma.[167] Initially remaining close to the water's edge, mutations and variations resulted in further colonization of this new environment. The timing of the first animals to leave the oceans is not precisely known: the oldest clear evidence is of arthropods on land around 450 Ma,[168] perhaps thriving and becoming better adapted due to the vast food source provided by the terrestrial plants. There is also unconfirmed evidence that arthropods may have appeared on land as early as 530 Ma.[169]
Evolution of tetrapods
At the end of the Ordovician period, 443 Ma,[22] additional extinction events occurred, perhaps due to a concurrent ice age.[154] Around 380 to 375 Ma, the first tetrapods evolved from fish.[170] Fins evolved to become limbs that the first tetrapods used to lift their heads out of the water to breathe air. This would let them live in oxygen-poor water, or pursue small prey in shallow water.[170] They may have later ventured on land for brief periods. Eventually, some of them became so well adapted to terrestrial life that they spent their adult lives on land, although they hatched in the water and returned to lay their eggs. This was the origin of the amphibians. About 365 Ma, another period of extinction occurred, perhaps as a result of global cooling.[171] Plants evolved seeds, which dramatically accelerated their spread on land, around this time (by approximately 360 Ma).[172][173]
About 20 million years later (340 Ma[123]: 293–296 ), the amniotic egg evolved, which could be laid on land, giving a survival advantage to tetrapod embryos. This resulted in the divergence of amniotes from amphibians. Another 30 million years (310 Ma[123]: 254–256 ) saw the divergence of the synapsids (including mammals) from the sauropsids (including birds and reptiles). Other groups of organisms continued to evolve, and lines diverged—in fish, insects, bacteria, and so on—but less is known of the details.[citation needed]
After yet another, the most severe extinction of the period (251~250 Ma), around 230 Ma, dinosaurs split off from their reptilian ancestors.[174] The Triassic–Jurassic extinction event at 200 Ma spared many of the dinosaurs,[22][175] and they soon became dominant among the vertebrates. Though some mammalian lines began to separate during this period, existing mammals were probably small animals resembling shrews.[123]: 169
The boundary between avian and non-avian dinosaurs is not clear, but Archaeopteryx, traditionally considered one of the first birds, lived around 150 Ma.[176]
The earliest evidence for the angiosperms evolving flowers is during the Cretaceous period, some 20 million years later (132 Ma).[177]
Extinctions
The first of five great mass extinctions was the Ordovician-Silurian extinction. Its possible cause was the intense glaciation of Gondwana, which eventually led to a snowball earth. 60% of marine invertebrates became extinct and 25% of all families.[citation needed]
The second mass extinction was the Late Devonian extinction, probably caused by the evolution of trees, which could have led to the depletion of greenhouse gases (like CO2) or the eutrophication of water. 70% of all species became extinct.[178]
The third mass extinction was the Permian-Triassic, or the Great Dying, event was possibly caused by some combination of the Siberian Traps volcanic event, an asteroid impact, methane hydrate gasification, sea level fluctuations, and a major anoxic event. Either the proposed Wilkes Land crater[179] in Antarctica or Bedout structure off the northwest coast of Australia may indicate an impact connection with the Permian-Triassic extinction. But it remains uncertain whether either these or other proposed Permian-Triassic boundary craters are either real impact craters or even contemporaneous with the Permian-Triassic extinction event. This was by far the deadliest extinction ever, with about 57% of all families and 83% of all genera killed.[180][181]
The fourth mass extinction was the Triassic-Jurassic extinction event in which almost all synapsids and archosaurs became extinct, probably due to new competition from dinosaurs.[182]
The fifth and most recent mass extinction was the Cretaceous-Paleogene extinction event. In 66 Ma, a 10-kilometer (6.2 mi) asteroid struck Earth just off the Yucatán Peninsula—somewhere in the southwestern tip of then Laurasia—where the Chicxulub crater is today. This ejected vast quantities of particulate matter and vapor into the air that occluded sunlight, inhibiting photosynthesis. 75% of all life, including the non-avian dinosaurs, became extinct,[183] marking the end of the Cretaceous period and Mesozoic era.[citation needed]
Diversification of mammals
The first true mammals evolved in the shadows of dinosaurs and other large archosaurs that filled the world by the late Triassic. The first mammals were very small, and were probably nocturnal to escape predation. Mammal diversification truly began only after the Cretaceous-Paleogene extinction event.[184] By the early Paleocene the earth recovered from the extinction, and mammalian diversity increased. Creatures like Ambulocetus took to the oceans to eventually evolve into whales,[185] whereas some creatures, like primates, took to the trees.[186] This all changed during the mid to late Eocene when the circum-Antarctic current formed between Antarctica and Australia which disrupted weather patterns on a global scale. Grassless savanna began to predominate much of the landscape, and mammals such as Andrewsarchus rose up to become the largest known terrestrial predatory mammal ever,[187] and early whales like Basilosaurus took control of the seas.[citation needed]
The evolution of grasses brought a remarkable change to the Earth's landscape, and the new open spaces created pushed mammals to get bigger and bigger. Grass started to expand in the Miocene, and the Miocene is where many modern- day mammals first appeared. Giant ungulates like Paraceratherium and Deinotherium evolved to rule the grasslands. The evolution of grass also brought primates down from the trees, and started human evolution. The first big cats evolved during this time as well.[188] The Tethys Sea was closed off by the collision of Africa and Europe.[189]
The formation of Panama was perhaps the most important geological event to occur in the last 60 million years. Atlantic and Pacific currents were closed off from each other, which caused the formation of the Gulf Stream, which made Europe warmer. The land bridge allowed the isolated creatures of South America to migrate over to North America, and vice versa.[190] Various species migrated south, leading to the presence in South America of llamas, the spectacled bear, kinkajous and jaguars.[citation needed]
Three million years ago saw the start of the Pleistocene epoch, which featured dramatic climatic changes due to the ice ages. The ice ages led to the evolution of modern man in Saharan Africa and expansion. The mega-fauna that dominated fed on grasslands that, by now, had taken over much of the subtropical world. The large amounts of water held in the ice allowed for various bodies of water to shrink and sometimes disappear such as the North Sea and the Bering Strait. It is believed by many that a huge migration took place along Beringia which is why, today, there are camels (which evolved and became extinct in North America), horses (which evolved and became extinct in North America), and Native Americans. The ending of the last ice age coincided with the expansion of man, along with a massive die out of ice age mega-fauna. This extinction is nicknamed "the Sixth Extinction".
Human evolution
−10 — – −9 — – −8 — – −7 — – −6 — – −5 — – −4 — – −3 — – −2 — – −1 — – 0 — |
| |||||||||||||||||||
A small African ape living around 6 Ma was the last animal whose descendants would include both modern humans and their closest relatives, the chimpanzees.[123]: 100–101 Only two branches of its family tree have surviving descendants. Very soon after the split, for reasons that are still unclear, apes in one branch developed the ability to walk upright.[123]: 95–99 Brain size increased rapidly, and by 2 Ma, the first animals classified in the genus Homo had appeared.[166]: 300 Of course, the line between different species or even genera is somewhat arbitrary as organisms continuously change over generations. Around the same time, the other branch split into the ancestors of the common chimpanzee and the ancestors of the bonobo as evolution continued simultaneously in all life forms.[123]: 100–101
The ability to control fire probably began in Homo erectus (or Homo ergaster), probably at least 790,000 years ago[191] but perhaps as early as 1.5 Ma.[123]: 67 The use and discovery of controlled fire may even predate Homo erectus. Fire was possibly used by the early Lower Paleolithic (Oldowan) hominid Homo habilis or strong australopithecines such as Paranthropus.[192]
It is more difficult to establish the origin of language; it is unclear whether Homo erectus could speak or if that capability had not begun until Homo sapiens.[123]: 67 As brain size increased, babies were born earlier, before their heads grew too large to pass through the pelvis. As a result, they exhibited more plasticity, and thus possessed an increased capacity to learn and required a longer period of dependence. Social skills became more complex, language became more sophisticated, and tools became more elaborate. This contributed to further cooperation and intellectual development.[194]: 7 Modern humans (Homo sapiens) are believed to have originated around 200,000 years ago or earlier in Africa; the oldest fossils date back to around 160,000 years ago.[195]
The first humans to show signs of spirituality are the Neanderthals (usually classified as a separate species with no surviving descendants); they buried their dead, often with no sign of food or tools.[196]: 17 However, evidence of more sophisticated beliefs, such as the early Cro-Magnon cave paintings (probably with magical or religious significance)[196]: 17–19 did not appear until 32,000 years ago.[197] Cro-Magnons also left behind stone figurines such as Venus of Willendorf, probably also signifying religious belief.[196]: 17–19 By 11,000 years ago, Homo sapiens had reached the southern tip of South America, the last of the uninhabited continents (except for Antarctica, which remained undiscovered until 1820 AD).[198] Tool use and communication continued to improve, and interpersonal relationships became more intricate.[citation needed]
Human history
Throughout more than 90% of its history, Homo sapiens lived in small bands as nomadic hunter-gatherers.[194]: 8 As language became more complex, the ability to remember and communicate information resulted, according to a theory proposed by Richard Dawkins, in a new replicator: the meme.[199] Ideas could be exchanged quickly and passed down the generations. Cultural evolution quickly outpaced biological evolution, and history proper began. Between 8500 and 7000 BC, humans in the Fertile Crescent in the Middle East began the systematic husbandry of plants and animals: agriculture.[200] This spread to neighboring regions, and developed independently elsewhere, until most Homo sapiens lived sedentary lives in permanent settlements as farmers. Not all societies abandoned nomadism, especially those in isolated areas of the globe poor in domesticable plant species, such as Australia.[201] However, among those civilizations that did adopt agriculture, the relative stability and increased productivity provided by farming allowed the population to expand.[citation needed]
Agriculture had a major impact; humans began to affect the environment as never before. Surplus food allowed a priestly or governing class to arise, followed by increasing division of labor. This led to Earth's first civilization at Sumer in the Middle East, between 4000 and 3000 BC.[194]: 15 Additional civilizations quickly arose in ancient Egypt, at the Indus River valley and in China. The invention of writing enabled complex societies to arise: record-keeping and libraries served as a storehouse of knowledge and increased the cultural transmission of information. Humans no longer had to spend all their time working for survival, enabling the first specialized occupations (e.g. craftsmen, merchants, priests, etc.). Curiosity and education drove the pursuit of knowledge and wisdom, and various disciplines, including science (in a primitive form), arose. This in turn led to the emergence of increasingly larger and more complex civilizations, such as the first empires, which at times traded with one another, or fought for territory and resources.
By around 500 BC, there were advanced civilizations in the Middle East, Iran, India, China, and Greece, at times expanding, at times entering into decline.[194]: 3 In 221 BC, China became a single polity that would grow to spread its culture throughout East Asia, and it has remained the most populous nation in the world. During this period, famous Hindu texts known as vedas came in existence in Indus valley civilization. This civilization developed in warfare, arts, science, mathematics and in architect.[citation needed] The fundamentals of Western civilization were largely shaped in Ancient Greece, with the world's first democratic government and major advances in philosophy and science, and in Ancient Rome with advances in law, government, and engineering.[202] The Roman Empire was Christianized by Emperor Constantine in the early 4th century and declined by the end of the 5th. Beginning with the 7th century, Christianization of Europe began, and since at least the 4th century Christianity has played a prominent role in the shaping of Western civilization.[203][204][205][206][207][208][209][210] In 610, Islam was founded and quickly became the dominant religion in Western Asia. The House of Wisdom was established in Abbasid-era Baghdad, Iraq.[211] It is considered to have been a major intellectual center during the Islamic Golden Age, where Muslim scholars in Baghdad and Cairo flourished from the ninth to the thirteenth centuries until the Mongol sack of Baghdad in 1258 AD. In 1054 AD the Great Schism between the Roman Catholic Church and the Eastern Orthodox Church led to the prominent cultural differences between Western and Eastern Europe.[212]
In the 14th century, the Renaissance began in Italy with advances in religion, art, and science.[194]: 317–319 At that time the Christian Church as a political entity lost much of its power. In 1492, Christopher Columbus reached the Americas, initiating great changes to the new world. European civilization began to change beginning in 1500, leading to the scientific and industrial revolutions. That continent began to exert political and cultural dominance over human societies around the world, a time known as the Colonial era (also see Age of Discovery).[194]: 295–299
Recent events
Change has continued at a rapid pace from the mid-1940s to today. Technological developments include nuclear weapons, computers, genetic engineering, and nanotechnology. Economic globalization, spurred by advances in communication and transportation technology, has influenced everyday life in many parts of the world. Cultural and institutional forms such as democracy, capitalism, and environmentalism have increased influence. Major concerns and problems such as disease, war, poverty, violent radicalism, and recently, human-caused climate change have risen as the world population increases.[citation needed]
In 1957, the Soviet Union launched the first artificial satellite into orbit and, soon afterward, Yuri Gagarin became the first human in space. Neil Armstrong, an American, was the first to set foot on another astronomical object, the Moon. Unmanned probes have been sent to all the known planets in the Solar System, with some (such as the two Voyager spacecraft) having left the Solar System. Five space agencies, representing over fifteen countries,[213] have worked together to build the International Space Station. Aboard it, there has been a continuous human presence in space since 2000.[214] The World Wide Web became a part of everyday life in the 1990s, and since then has become an indispensable source of information in the developed world.[citation needed]
See also
- Chronology of the universe – History and future of the universe
- Detailed logarithmic timeline – Timeline of the history of the universe, Earth, and mankind
- Earth phase – Phases of the Earth as seen from the Moon
- Evolutionary history of life
- Future of Earth – Long-term extrapolated geological and biological changes of Planet Earth
- Geological history of Earth – The sequence of major geological events in Earth's past
- Global catastrophic risk – Potentially harmful worldwide events
- Timeline of the evolutionary history of life – Major events during the development of life
- Timeline of natural history
https://en.wikipedia.org/wiki/History_of_Earth#Tectonics,_paleogeography_and_climate
The Paleocene, ( /ˈpæli.əsiːn, -i.oʊ-, ˈpeɪli-/ PAL-ee-ə-seen, -ee-oh-, PAY-lee-)[4] or Palaeocene, is a geological epoch that lasted from about 66 to 56 million years ago (mya). It is the first epoch of the Paleogene Period in the modern Cenozoic Era. The name is a combination of the Ancient Greek παλαιός palaiós meaning "old" and the Eocene Epoch (which succeeds the Paleocene), translating to "the old part of the Eocene".
The epoch is bracketed by two major events in Earth's history. The K–Pg extinction event, brought on by an asteroid impact and possibly volcanism, marked the beginning of the Paleocene and killed off 75% of living species, most famously the non-avian dinosaurs. The end of the epoch was marked by the Paleocene–Eocene Thermal Maximum (PETM), which was a major climatic event wherein about 2,500–4,500 gigatons of carbon were released into the atmosphere and ocean systems, causing a spike in global temperatures and ocean acidification.
In the Paleocene, the continents of the Northern Hemisphere were still connected via some land bridges; and South America, Antarctica, and Australia had not completely separated yet. The Rocky Mountains were being uplifted, the Americas had not yet joined, the Indian Plate had begun its collision with Asia, and the North Atlantic Igneous Province was forming in the third-largest magmatic event of the last 150 million years. In the oceans, the thermohaline circulation probably was much different from what it is today, with downwellings occurring in the North Pacific rather than the North Atlantic, and water density mainly being controlled by salinity rather than temperature.
The K–Pg extinction event caused a floral and faunal turnover of species, with previously abundant species being replaced by previously uncommon ones. In the Paleocene, with a global average temperature of about 24–25 °C (75–77 °F), compared to 14 °C (57 °F) in more recent times, the Earth had a greenhouse climate without permanent ice sheets at the poles, like the preceding Mesozoic. As such, there were forests worldwide—including at the poles—but they had low species richness in regards to plant life, and were populated by mainly small creatures that were rapidly evolving to take advantage of the recently emptied Earth. Though some animals attained great size, most remained rather small. The forests grew quite dense in the general absence of large herbivores. Mammals proliferated in the Paleocene, and the earliest placental and marsupial mammals are recorded from this time, but most Paleocene taxa have ambiguous affinities. In the seas, ray-finned fish rose to dominate open ocean and reef ecosystems.
https://en.wikipedia.org/wiki/Paleocene
The Paleozoic (or Palaeozoic) Era is the earliest of three geologic eras of the Phanerozoic Eon. The name Paleozoic ( /ˌpæli.əˈzoʊ.ɪk, -i.oʊ-, ˌpeɪ-/ pal-ee-ə-ZOH-ik, -ee-oh-, pay-;[1][2]) was coined by the British geologist Adam Sedgwick in 1838[3] by combining the Greek words palaiós (παλαιός, "old") and zōḗ (ζωή), "life", meaning "ancient life"[4]).
It is the longest of the Phanerozoic eras, lasting from 538.8 to 251.902 million years ago, and is subdivided into six geologic periods (from oldest to youngest):
The Paleozoic comes after the Neoproterozoic Era of the Proterozoic Eon and is followed by the Mesozoic Era.
The Paleozoic was a time of dramatic geological, climatic, and evolutionary change. The Cambrian witnessed the most rapid and widespread diversification of life in Earth's history, known as the Cambrian explosion, in which most modern phyla first appeared. Arthropods, molluscs, fish, amphibians, reptiles, and synapsids all evolved during the Paleozoic. Life began in the ocean but eventually transitioned onto land, and by the late Paleozoic, great forests of primitive plants covered the continents, many of which formed the coal beds of Europe and eastern North America. Towards the end of the era, large, sophisticated synapsids and diapsids were dominant and the first modern plants (conifers) appeared.
The Paleozoic Era ended with the largest extinction event of the Phanerozoic Eon,[a] the Permian–Triassic extinction event. The effects of this catastrophe were so devastating that it took life on land 30 million years into the Mesozoic Era to recover.[5] Recovery of life in the sea may have been much faster.[6]
Geology
The Paleozoic Era began with the breakup of the supercontinent of Pannotia[7][8] and ended with the assembly of the supercontinent of Pangaea.[9] The breakup of Pannotia began with the opening of the Iapetus Ocean and other Cambrian seas and coincided with a dramatic rise in sea level.[10] Paleoclimatic studies and evidence of glaciers indicate that Central Africa was most likely in the polar regions during the early Paleozoic. The breakup of Pannotia was followed by the assembly of the huge continent Gondwana (510 million years ago). By mid-Paleozoic, the collision of North America and Europe produced the Acadian-Caledonian uplifts, and a subduction plate uplifted eastern Australia. By the late Paleozoic, continental collisions formed the supercontinent of Pangaea and created great mountain chains, including the Appalachians, Ural Mountains, and mountains of Tasmania.[9]
Periods of the Paleozoic Era
There are six periods in the Paleozoic Era: Cambrian, Ordovician, Silurian, Devonian, Carboniferous (subdivided into the Mississippian and the Pennsylvanian subperiods), and the Permian.[11]
Cambrian Period
The Cambrian spanned from 539–485 million years ago and is the first period of the Paleozoic Era of the Phanerozoic. The Cambrian marked a boom in evolution in an event known as the Cambrian explosion in which the largest number of creatures evolved in any single period of the history of the Earth. Creatures like algae evolved, but the most ubiquitous of that period were the armored arthropods, like trilobites. Almost all marine phyla evolved in this period. During this time, the supercontinent Pannotia begins to break up, most of which later became the supercontinent Gondwana.[12]
Ordovician Period
The Ordovician spanned from 485–444 million years ago. The Ordovician was a time in Earth's history in which many of the biological classes still prevalent today evolved, such as primitive fish, cephalopods, and coral. The most common forms of life, however, were trilobites, snails and shellfish. The first arthropods went ashore to colonize the empty continent of Gondwana. By the end of the Ordovician, Gondwana was at the south pole, early North America had collided with Europe, closing the Atlantic Ocean. Glaciation of Africa resulted in a major drop in sea level, killing off all life that had established along coastal Gondwana. Glaciation may have caused the Ordovician–Silurian extinction events, in which 60% of marine invertebrates and 25% of families became extinct, and is considered the first Phanerozoic mass extinction event, and the second deadliest.[a][13]
Silurian Period
The Silurian spanned from 444–419 million years ago. The Silurian saw the rejuvenation of life as the Earth recovered from the previous glaciation. This period saw the mass evolution of fish, as jawless fish became more numerous, jawed fish evolved, and the first freshwater fish evolved, though arthropods, such as sea scorpions, were still apex predators. Fully terrestrial life evolved, including early arachnids, fungi, and centipedes. The evolution of vascular plants (Cooksonia) allowed plants to gain a foothold on land. These early plants were the forerunners of all plant life on land. During this time, there were four continents: Gondwana (Africa, South America, Australia, Antarctica, Siberia), Laurentia (North America), Baltica (Northern Europe), and Avalonia (Western Europe). The recent rise in sea levels allowed many new species to thrive in water.[14]
Devonian Period
The Devonian spanned from 419–359 million years ago. Also known as "The Age of the Fish", the Devonian featured a huge diversification of fish, including armored fish like Dunkleosteus and lobe-finned fish which eventually evolved into the first tetrapods. On land, plant groups diversified incredibly in an event known as the Devonian explosion when plants made lignin allowing taller growth and vascular tissue: the first trees evolved, as well as seeds. This event also diversified arthropod life, by providing them new habitats. The first amphibians also evolved, and the fish were now at the top of the food chain. Near the end of the Devonian, 70% of all species became extinct in an event known as the Late Devonian extinction, which was the Earth's second Phanerozoic mass extinction event.[a][15]
Carboniferous Period
The Carboniferous spanned from 359–299 million years ago. During this time, average global temperatures were exceedingly high; the early Carboniferous averaged at about 20 degrees Celsius (but cooled to 10 °C during the Middle Carboniferous).[16] Tropical swamps dominated the Earth, and the lignin stiffened trees grew to greater heights and number. As the bacteria and fungi capable of eating the lignin had not yet evolved, their remains were left buried, which created much of the carbon that became the coal deposits of today (hence the name "Carboniferous"). Perhaps the most important evolutionary development of the time was the evolution of amniotic eggs, which allowed amphibians to move farther inland and remain the dominant vertebrates for the duration of this period. Also, the first reptiles and synapsids evolved in the swamps. Throughout the Carboniferous, there was a cooling trend, which led to the Permo-Carboniferous glaciation or the Carboniferous Rainforest Collapse. Gondwana was glaciated as much of it was situated around the south pole.[17]
Permian Period
The Permian spanned from 299–252 million years ago and was the last period of the Paleozoic Era. At the beginning of this period, all continents joined together to form the supercontinent Pangaea, which was encircled by one ocean called Panthalassa. The land mass was very dry during this time, with harsh seasons, as the climate of the interior of Pangaea was not regulated by large bodies of water. Diapsids and synapsids flourished in the new dry climate. Creatures such as Dimetrodon and Edaphosaurus ruled the new continent. The first conifers evolved, and dominated the terrestrial landscape. Near the end of the Permian, however, Pangaea grew drier. The interior was desert, and new taxa such as Scutosaurus and Gorgonopsids filled it. Eventually they disappeared, along with 95% of all life on Earth, in a cataclysm known as "The Great Dying", the third and most severe Phanerozoic mass extinction.[a][18][19]
Climate
The early Cambrian climate was probably moderate at first, becoming warmer over the course of the Cambrian, as the second-greatest sustained sea level rise in the Phanerozoic got underway. However, as if to offset this trend, Gondwana moved south, so that, in Ordovician time, most of West Gondwana (Africa and South America) lay directly over the South Pole.
The early Paleozoic climate was strongly zonal, with the result that the "climate", in an abstract sense, became warmer, but the living space of most organisms of the time – the continental shelf marine environment – became steadily colder. However, Baltica (Northern Europe and Russia) and Laurentia (eastern North America and Greenland) remained in the tropical zone, while China and Australia lay in waters which were at least temperate. The early Paleozoic ended, rather abruptly, with the short, but apparently severe, late Ordovician ice age. This cold spell caused the second-greatest mass extinction of the Phanerozoic Eon.[a] Over time, the warmer weather moved into the Paleozoic Era.
The Ordovician and Silurian were warm greenhouse periods, with the highest sea levels of the Paleozoic (200 m above today's); the warm climate was interrupted only by a 30 million year cool period, the Early Palaeozoic Icehouse, culminating in the Hirnantian glaciation, 445 million years ago at the end of the Ordovician.[20]
The middle Paleozoic was a time of considerable stability. Sea levels had dropped coincident with the ice age, but slowly recovered over the course of the Silurian and Devonian. The slow merger of Baltica and Laurentia, and the northward movement of bits and pieces of Gondwana created numerous new regions of relatively warm, shallow sea floor. As plants took hold on the continental margins, oxygen levels increased and carbon dioxide dropped, although much less dramatically. The north–south temperature gradient also seems to have moderated, or metazoan life simply became hardier, or both. At any event, the far southern continental margins of Antarctica and West Gondwana became increasingly less barren. The Devonian ended with a series of turnover pulses which killed off much of middle Paleozoic vertebrate life, without noticeably reducing species diversity overall.
There are many unanswered questions about the late Paleozoic. The Mississippian (early Carboniferous Period) began with a spike in atmospheric oxygen, while carbon dioxide plummeted to new lows. This destabilized the climate and led to one, and perhaps two, ice ages during the Carboniferous. These were far more severe than the brief Late Ordovician ice age; but, this time, the effects on world biota were inconsequential. By the Cisuralian Epoch, both oxygen and carbon dioxide had recovered to more normal levels. On the other hand, the assembly of Pangaea created huge arid inland areas subject to temperature extremes. The Lopingian Epoch is associated with falling sea levels, increased carbon dioxide and general climatic deterioration, culminating in the devastation of the Permian extinction.
Flora
While macroscopic plant life appeared early in the Paleozoic Era and possibly late in the Neoproterozoic Era of the earlier eon, plants mostly remained aquatic until the Silurian Period, about 420 million years ago, when they began to transition onto dry land. Terrestrial flora reached its climax in the Carboniferous, when towering lycopsid rainforests dominated the tropical belt of Euramerica. Climate change caused the Carboniferous Rainforest Collapse which fragmented this habitat, diminishing the diversity of plant life in the late Carboniferous and Permian periods.[21]
Fauna
A noteworthy feature of Paleozoic life is the sudden appearance of nearly all of the invertebrate animal phyla in great abundance at the beginning of the Cambrian. The first vertebrates appeared in the form of primitive fish, which greatly diversified in the Silurian and Devonian Periods. The first animals to venture onto dry land were the arthropods. Some fish had lungs, and powerful bony fins that in the late Devonian, 367.5 million years ago, allowed them to crawl onto land. The bones in their fins eventually evolved into legs and they became the first tetrapods, 390 million years ago, and began to develop lungs. Amphibians were the dominant tetrapods until the mid-Carboniferous, when climate change greatly reduced their diversity. Later, reptiles prospered and continued to increase in number and variety by the late Permian period.[21]
See also
- Geologic time scale – System that relates geologic strata to time
- Precambrian – History of Earth 4600–539 million years ago
- Cenozoic – Third era of the Phanerozoic Eon (66 million years ago to present)
- Mesozoic – Second era of the Phanerozoic Eon: ~252–66 million years ago
- Phanerozoic – Fourth and current eon of the geological timescale
Footnotes
https://en.wikipedia.org/wiki/Paleozoic
An extinction event (also known as a mass extinction or biotic crisis) is a widespread and rapid decrease in the biodiversity on Earth. Such an event is identified by a sharp change in the diversity and abundance of multicellular organisms. It occurs when the rate of extinction increases with respect to the background extinction rate[1] and the rate of speciation. Estimates of the number of major mass extinctions in the last 540 million years range from as few as five to more than twenty. These differences stem from disagreement as to what constitutes a "major" extinction event, and the data chosen to measure past diversity.
https://en.wikipedia.org/wiki/Extinction_event
Identifying causes of specific mass extinctions
A good theory for a particular mass extinction should:
- explain all of the losses, not just focus on a few groups (such as dinosaurs);
- explain why particular groups of organisms died out and why others survived;
- provide mechanisms that are strong enough to cause a mass extinction but not a total extinction;
- be based on events or processes that can be shown to have happened, not just inferred from the extinction.
It may be necessary to consider combinations of causes. For example, the marine aspect of the end-Cretaceous extinction appears to have been caused by several processes that partially overlapped in time and may have had different levels of significance in different parts of the world.[92]
Arens and West (2006) proposed a "press / pulse" model in which mass extinctions generally require two types of cause: long-term pressure on the eco-system ("press") and a sudden catastrophe ("pulse") towards the end of the period of pressure.[93] Their statistical analysis of marine extinction rates throughout the Phanerozoic suggested that neither long-term pressure alone nor a catastrophe alone was sufficient to cause a significant increase in the extinction rate.
Most widely supported explanations
MacLeod (2001)[94] summarized the relationship between mass extinctions and events that are most often cited as causes of mass extinctions, using data from Courtillot, Jaeger & Yang et al. (1996),[95] Hallam (1992)[96] and Grieve & Pesonen (1992): [97]
- Flood basalt events (giant volcanic eruptions): 11 occurrences, all associated with significant extinctions[d][e] But Wignall (2001) concluded that only five of the major extinctions coincided with flood basalt eruptions and that the main phase of extinctions started before the eruptions.[98]
- Sea-level falls: 12, of which seven were associated with significant extinctions.[e]
- Asteroid impacts: one large impact is associated with a mass extinction, that is, the Cretaceous–Paleogene extinction event; there have been many smaller impacts but they are not associated with significant extinctions,[99] or cannot be dated precisely enough. The impact that created the Siljan Ring either was just before the Late Devonian Extinction or coincided with it.[100]
The most commonly suggested causes of mass extinctions are listed below.
Flood basalt events
The formation of large igneous provinces by flood basalt events could have:
- produced dust and particulate aerosols, which inhibited photosynthesis and thus caused food chains to collapse both on land and at sea[101]
- emitted sulfur oxides that were precipitated as acid rain and poisoned many organisms, contributing further to the collapse of food chains
- emitted carbon dioxide and thus possibly causing sustained global warming once the dust and particulate aerosols dissipated.
Flood basalt events occur as pulses of activity punctuated by dormant periods. As a result, they are likely to cause the climate to oscillate between cooling and warming, but with an overall trend towards warming as the carbon dioxide they emit can stay in the atmosphere for hundreds of years.
It is speculated that massive volcanism caused or contributed to the End-Permian, End-Triassic and End-Cretaceous extinctions.[102] The correlation between gigantic volcanic events expressed in the large igneous provinces and mass extinctions was shown for the last 260 million years.[103][104] Recently such possible correlation was extended across the whole Phanerozoic Eon.[105]
Sea-level fall
These are often clearly marked by worldwide sequences of contemporaneous sediments that show all or part of a transition from sea-bed to tidal zone to beach to dry land – and where there is no evidence that the rocks in the relevant areas were raised by geological processes such as orogeny. Sea-level falls could reduce the continental shelf area (the most productive part of the oceans) sufficiently to cause a marine mass extinction, and could disrupt weather patterns enough to cause extinctions on land. But sea-level falls are very probably the result of other events, such as sustained global cooling or the sinking of the mid-ocean ridges.
Sea-level falls are associated with most of the mass extinctions, including all of the "Big Five"—End-Ordovician, Late Devonian, End-Permian, End-Triassic, and End-Cretaceous, along with the more recently recognised Capitanian mass extinction of comparable severity to the Big Five.[106][107]
A 2008 study, published in the journal Nature, established a relationship between the speed of mass extinction events and changes in sea level and sediment.[108] The study suggests changes in ocean environments related to sea level exert a driving influence on rates of extinction, and generally determine the composition of life in the oceans.[109]
Extraterrestrial threats
Impact events
The impact of a sufficiently large asteroid or comet could have caused food chains to collapse both on land and at sea by producing dust and particulate aerosols and thus inhibiting photosynthesis.[110] Impacts on sulfur-rich rocks could have emitted sulfur oxides precipitating as poisonous acid rain, contributing further to the collapse of food chains. Such impacts could also have caused megatsunamis and/or global forest fires.
Most paleontologists now agree that an asteroid did hit the Earth about 66 Ma, but there is lingering dispute whether the impact was the sole cause of the Cretaceous–Paleogene extinction event.[111][112]
Nonetheless, in October 2019, researchers reported that the Cretaceous Chicxulub asteroid impact that resulted in the extinction of non-avian dinosaurs 66 Ma, also rapidly acidified the oceans, producing ecological collapse and long-lasting effects on the climate, and was a key reason for end-Cretaceous mass extinction.[113][114]
According to the Shiva Hypothesis, the Earth is subject to increased asteroid impacts about once every 27 million years because of the Sun's passage through the plane of the Milky Way galaxy, thus causing extinction events at 27 million year intervals. Some evidence for this hypothesis has emerged in both marine and non-marine contexts.[115] Alternatively, the Sun's passage through the higher density spiral arms of the galaxy could coincide with mass extinction on Earth, perhaps due to increased impact events.[116] However, a reanalysis of the effects of the Sun's transit through the spiral structure based on maps of the spiral structure of the Milky Way in CO molecular line emission has failed to find a correlation.[117]
A nearby nova, supernova or gamma ray burst
A nearby gamma-ray burst (less than 6000 light-years away) would be powerful enough to destroy the Earth's ozone layer, leaving organisms vulnerable to ultraviolet radiation from the Sun.[118] Gamma ray bursts are fairly rare, occurring only a few times in a given galaxy per million years.[119] It has been suggested that a supernova or gamma ray burst caused the End-Ordovician extinction.[120]
Global cooling
Sustained and significant global cooling could kill many polar and temperate species and force others to migrate towards the equator; reduce the area available for tropical species; often make the Earth's climate more arid on average, mainly by locking up more of the planet's water in ice and snow. The glaciation cycles of the current ice age are believed to have had only a very mild impact on biodiversity, so the mere existence of a significant cooling is not sufficient on its own to explain a mass extinction.
It has been suggested that global cooling caused or contributed to the End-Ordovician, Permian–Triassic, Late Devonian extinctions, and possibly others. Sustained global cooling is distinguished from the temporary climatic effects of flood basalt events or impacts.
Global warming
This would have the opposite effects: expand the area available for tropical species; kill temperate species or force them to migrate towards the poles; possibly cause severe extinctions of polar species; often make the Earth's climate wetter on average, mainly by melting ice and snow and thus increasing the volume of the water cycle. It might also cause anoxic events in the oceans (see below).
Global warming as a cause of mass extinction is supported by several recent studies.[121]
The most dramatic example of sustained warming is the Paleocene–Eocene Thermal Maximum, which was associated with one of the smaller mass extinctions. It has also been suggested to have caused the Triassic–Jurassic extinction event, during which 20% of all marine families became extinct. Furthermore, the Permian–Triassic extinction event has been suggested to have been caused by warming.[122][123][124]
Clathrate gun hypothesis
Clathrates are composites in which a lattice of one substance forms a cage around another. Methane clathrates (in which water molecules are the cage) form on continental shelves. These clathrates are likely to break up rapidly and release the methane if the temperature rises quickly or the pressure on them drops quickly—for example in response to sudden global warming or a sudden drop in sea level or even earthquakes. Methane is a much more powerful greenhouse gas than carbon dioxide, so a methane eruption ("clathrate gun") could cause rapid global warming or make it much more severe if the eruption was itself caused by global warming.
The most likely signature of such a methane eruption would be a sudden decrease in the ratio of carbon-13 to carbon-12 in sediments, since methane clathrates are low in carbon-13; but the change would have to be very large, as other events can also reduce the percentage of carbon-13.[125]
It has been suggested that "clathrate gun" methane eruptions were involved in the end-Permian extinction ("the Great Dying") and in the Paleocene–Eocene Thermal Maximum, which was associated with one of the smaller mass extinctions.
Anoxic events
Anoxic events are situations in which the middle and even the upper layers of the ocean become deficient or totally lacking in oxygen. Their causes are complex and controversial, but all known instances are associated with severe and sustained global warming, mostly caused by sustained massive volcanism.[126]
It has been suggested that anoxic events caused or contributed to the Ordovician–Silurian, late Devonian, Permian–Triassic and Triassic–Jurassic extinctions, as well as a number of lesser extinctions (such as the Ireviken, Mulde, Lau, Toarcian and Cenomanian–Turonian events). On the other hand, there are widespread black shale beds from the mid-Cretaceous that indicate anoxic events but are not associated with mass extinctions.
The bio-availability of essential trace elements (in particular selenium) to potentially lethal lows has been shown to coincide with, and likely have contributed to, at least three mass extinction events in the oceans, that is, at the end of the Ordovician, during the Middle and Late Devonian, and at the end of the Triassic. During periods of low oxygen concentrations very soluble selenate (Se6+) is converted into much less soluble selenide (Se2-), elemental Se and organo-selenium complexes. Bio-availability of selenium during these extinction events dropped to about 1% of the current oceanic concentration, a level that has been proven lethal to many extant organisms.[127]
British oceanologist and atmospheric scientist, Andrew Watson, explained that, while the Holocene epoch exhibits many processes reminiscent of those that have contributed to past anoxic events, full-scale ocean anoxia would take "thousands of years to develop".[128]
Hydrogen sulfide emissions from the seas
Kump, Pavlov and Arthur (2005) have proposed that during the Permian–Triassic extinction event the warming also upset the oceanic balance between photosynthesising plankton and deep-water sulfate-reducing bacteria, causing massive emissions of hydrogen sulfide, which poisoned life on both land and sea and severely weakened the ozone layer, exposing much of the life that still remained to fatal levels of UV radiation.[129][130][15]
Oceanic overturn
Oceanic overturn is a disruption of thermo-haline circulation that lets surface water (which is more saline than deep water because of evaporation) sink straight down, bringing anoxic deep water to the surface and therefore killing most of the oxygen-breathing organisms that inhabit the surface and middle depths. It may occur either at the beginning or the end of a glaciation, although an overturn at the start of a glaciation is more dangerous because the preceding warm period will have created a larger volume of anoxic water.[131]
Unlike other oceanic catastrophes such as regressions (sea-level falls) and anoxic events, overturns do not leave easily identified "signatures" in rocks and are theoretical consequences of researchers' conclusions about other climatic and marine events.
It has been suggested that oceanic overturn caused or contributed to the late Devonian and Permian–Triassic extinctions.
Geomagnetic reversal
One theory is that periods of increased geomagnetic reversals will weaken Earth's magnetic field long enough to expose the atmosphere to the solar winds, causing oxygen ions to escape the atmosphere in a rate increased by 3–4 orders, resulting in a disastrous decrease in oxygen.[132]
Plate tectonics
Movement of the continents into some configurations can cause or contribute to extinctions in several ways: by initiating or ending ice ages; by changing ocean and wind currents and thus altering climate; by opening seaways or land bridges that expose previously isolated species to competition for which they are poorly adapted (for example, the extinction of most of South America's native ungulates and all of its large metatherians after the creation of a land bridge between North and South America). Occasionally continental drift creates a super-continent that includes the vast majority of Earth's land area, which in addition to the effects listed above is likely to reduce the total area of continental shelf (the most species-rich part of the ocean) and produce a vast, arid continental interior that may have extreme seasonal variations.
Another theory is that the creation of the super-continent Pangaea contributed to the End-Permian mass extinction. Pangaea was almost fully formed at the transition from mid-Permian to late-Permian, and the "Marine genus diversity" diagram at the top of this article shows a level of extinction starting at that time, which might have qualified for inclusion in the "Big Five" if it were not overshadowed by the "Great Dying" at the end of the Permian.[133]
Other hypotheses
Many other hypotheses have been proposed, such as the spread of a new disease, or simple out-competition following an especially successful biological innovation. But all have been rejected, usually for one of the following reasons: they require events or processes for which there is no evidence; they assume mechanisms that are contrary to the available evidence; they are based on other theories that have been rejected or superseded.
Scientists have been concerned that human activities could cause more plants and animals to become extinct than any point in the past. Along with human-made changes in climate (see above), some of these extinctions could be caused by overhunting, overfishing, invasive species, or habitat loss. A study published in May 2017 in Proceedings of the National Academy of Sciences argued that a “biological annihilation” akin to a sixth mass extinction event is underway as a result of anthropogenic causes, such as over-population and over-consumption. The study suggested that as much as 50% of the number of animal individuals that once lived on Earth were already extinct, threatening the basis for human existence too.[134][30]
Future biosphere extinction/sterilization
The eventual warming and expanding of the Sun, combined with the eventual decline of atmospheric carbon dioxide, could actually cause an even greater mass extinction, having the potential to wipe out even microbes (in other words, the Earth would be completely sterilized): rising global temperatures caused by the expanding Sun would gradually increase the rate of weathering, which would in turn remove more and more CO2 from the atmosphere. When CO2 levels get too low (perhaps at 50 ppm), most plant life will die out, although simpler plants like grasses and mosses can survive much longer, until CO2 levels drop to 10 ppm.[135][136]
With all photosynthetic organisms gone, atmospheric oxygen can no longer be replenished, and it is eventually removed by chemical reactions in the atmosphere, perhaps from volcanic eruptions. Eventually the loss of oxygen will cause all remaining aerobic life to die out via asphyxiation, leaving behind only simple anaerobic prokaryotes. When the Sun becomes 10% brighter in about a billion years,[135] Earth will suffer a moist greenhouse effect resulting in its oceans boiling away, while the Earth's liquid outer core cools due to the inner core's expansion and causes the Earth's magnetic field to shut down. In the absence of a magnetic field, charged particles from the Sun will deplete the atmosphere and further increase the Earth's temperature to an average of around 420 K (147 °C, 296 °F) in 2.8 billion years, causing the last remaining life on Earth to die out. This is the most extreme instance of a climate-caused extinction event. Since this will only happen late in the Sun's life, it would represent the final mass extinction in Earth's history (albeit a very long extinction event).[135][136]
Effects and recovery
The effects of mass extinction events varied widely. After a major extinction event, usually only weedy species survive due to their ability to live in diverse habitats.[137] Later, species diversify and occupy empty niches. Generally, it takes millions of years for biodiversity to recover after extinction events.[138] In the most severe mass extinctions it may take 15 to 30 million years.[137]
The worst Phanerozoic event, the Permian–Triassic extinction, devastated life on Earth, killing over 90% of species. Life seemed to recover quickly after the P-T extinction, but this was mostly in the form of disaster taxa, such as the hardy Lystrosaurus. The most recent research indicates that the specialized animals that formed complex ecosystems, with high biodiversity, complex food webs and a variety of niches, took much longer to recover. It is thought that this long recovery was due to successive waves of extinction that inhibited recovery, as well as prolonged environmental stress that continued into the Early Triassic. Recent research indicates that recovery did not begin until the start of the mid-Triassic, four to six million years after the extinction;[139] and some writers estimate that the recovery was not complete until 30 million years after the P-T extinction, that is, in the late Triassic.[140] Subsequent to the P-T extinction, there was an increase in provincialization, with species occupying smaller ranges – perhaps removing incumbents from niches and setting the stage for an eventual rediversification.[141]
The effects of mass extinctions on plants are somewhat harder to quantify, given the biases inherent in the plant fossil record. Some mass extinctions (such as the end-Permian) were equally catastrophic for plants, whereas others, such as the end-Devonian, did not affect the flora.[142]
See also
- Bioevent
- Elvis taxon
- Endangered species
- Geologic time scale
- Global catastrophic risk
- Holocene extinction
- Human extinction
- Kačák Event
- Lazarus taxon
- List of impact craters on Earth
- List of largest volcanic eruptions
- List of possible impact structures on Earth
- Medea hypothesis
- Rare species
- Signor–Lipps effect
- Snowball Earth
- Speculative evolution
- The Sixth Extinction: An Unnatural History (nonfiction book)
- Timeline of extinctions in the Holocene
Hydrogen sulfide emissions from the seas
Kump, Pavlov and Arthur (2005) have proposed that during the Permian–Triassic extinction event the warming also upset the oceanic balance between photosynthesising plankton and deep-water sulfate-reducing bacteria, causing massive emissions of hydrogen sulfide, which poisoned life on both land and sea and severely weakened the ozone layer, exposing much of the life that still remained to fatal levels of UV radiation.[129][130][15]
https://en.wikipedia.org/wiki/Extinction_event#Identifying_causes_of_specific_mass_extinctions
Sulfate-reducing microorganisms (SRM) or sulfate-reducing prokaryotes (SRP) are a group composed of sulfate-reducing bacteria (SRB) and sulfate-reducing archaea (SRA), both of which can perform anaerobic respiration utilizing sulfate (SO2−
4) as terminal electron acceptor, reducing it to hydrogen sulfide (H2S).[1][2] Therefore, these sulfidogenic microorganisms "breathe" sulfate rather than molecular oxygen (O2), which is the terminal electron acceptor reduced to water (H2O) in aerobic respiration.
https://en.wikipedia.org/wiki/Sulfate-reducing_microorganism
Jump to navigation Jump to searchLithotrophs are a diverse group of organisms using an inorganic substrate (usually of mineral origin) to obtain reducing equivalents for use in biosynthesis (e.g., carbon dioxide fixation) or energy conservation (i.e., ATP production) via aerobic or anaerobic respiration.[1] While lithotrophs in the broader sense include photolithotrophs like plants, chemolithotrophs are exclusively microorganisms; no known macrofauna possesses the ability to use inorganic compounds as electron sources. Macrofauna and lithotrophs can form symbiotic relationships, in which case the lithotrophs are called "prokaryotic symbionts". An example of this is chemolithotrophic bacteria in giant tube worms or plastids, which are organelles within plant cells that may have evolved from photolithotrophic cyanobacteria-like organisms. Chemolithotrophs belong to the domains Bacteria and Archaea. The term "lithotroph" was created from the Greek terms 'lithos' (rock) and 'troph' (consumer), meaning "eaters of rock". Many but not all lithoautotrophs are extremophiles.
The last universal common ancestor of life is thought to be a chemolithotroph (due to its presence in the prokaryotes).[2] Different from a lithotroph is an organotroph, an organism which obtains its reducing agents from the catabolism of organic compounds.
https://en.wikipedia.org/wiki/Lithotroph
https://en.wikipedia.org/wiki/Sulfate-reducing_microorganism
Biochemistry
Before sulfate can be used as an electron acceptor, it must be activated. This is done by the enzyme ATP-sulfurylase, which uses ATP and sulfate to create adenosine 5′-phosphosulfate (APS). APS is subsequently reduced to sulfite and AMP. Sulfite is then further reduced to sulfide, while AMP is turned into ADP using another molecule of ATP. The overall process, thus, involves an investment of two molecules of the energy carrier ATP, which must to be regained from the reduction.[1]
The enzyme dissimilatory (bi)sulfite reductase, dsrAB (EC 1.8.99.5), that catalyzes the last step of dissimilatory sulfate reduction, is the functional gene most used as a molecular marker to detect the presence of sulfate-reducing microorganisms.[18]
https://en.wikipedia.org/wiki/Sulfate-reducing_microorganism
Jump to navigation Jump to search
Quinone-interacting membrane-bound oxidoreductase is a membrane-bound protein complex present in the electron transport chain of sulfate reducers (e.g. Desulfovibrio species) and some sulfur oxidizers.
It was first described by Pires et al. (2003).[1]
https://en.wikipedia.org/wiki/Quinone-interacting_membrane-bound_oxidoreductase
https://en.wikipedia.org/wiki/Syntrophobacterales
https://en.wikipedia.org/wiki/Desulfobacterales
https://en.wikipedia.org/wiki/Desulfovibrionales
https://en.wikipedia.org/wiki/Thermodesulfobacteriota
https://en.wikipedia.org/wiki/Desulfotomaculum
https://en.wikipedia.org/wiki/Desulfosporomusa
https://en.wikipedia.org/wiki/Desulfosporosinus
https://en.wikipedia.org/wiki/Nitrospirota
https://en.wikipedia.org/wiki/Thermodesulfobium
https://en.wikipedia.org/wiki/Sulfate_adenylyltransferase
https://en.wikipedia.org/wiki/Adenosine_triphosphate
https://en.wikipedia.org/wiki/Prebiotic_atmosphere
https://en.wikipedia.org/wiki/Great_Oxidation_Event
https://en.wikipedia.org/wiki/Faint_young_Sun_paradox
https://en.wikipedia.org/wiki/Paleoproterozoic
https://en.wikipedia.org/wiki/Paleoarchean
https://en.wikipedia.org/wiki/Paleogene
Ypresian | |
---|---|
![]() Earth ~50 mya | |
https://en.wikipedia.org/wiki/Ypresian
https://en.wikipedia.org/wiki/Ypresian
Hadean rocks are very rare, largely consisting of zircons from one locality in Western Australia.[6] Hadean geophysical models remain controversial among geologists: it appears that plate tectonics and the growth of continents may have started in the Hadean.[6] Earth in the early Hadean had a very thick carbon dioxide atmosphere, but eventually oceans of liquid water formed.
References
- Dalrymple, G. Brent (2001). "The age of the Earth in the twentieth century: a problem (mostly) solved". Geological Society, London, Special Publications. 190 (1): 205–221. Bibcode:2001GSLSP.190..205D. doi:10.1144/gsl.sp.2001.190.01.14. S2CID 130092094. Retrieved 2022-10-02.
- "Age of the Earth". U.S. Geological Survey. 1997. Archived from the original on 23 December 2005. Retrieved 2022-10-03.
- Strachan, R.; Murphy, J.B.; Darling, J.; Storey, C.; Shields, G. (2020). "Precambrian (4.56–1 Ga)". In Gradstein, F.M.; Ogg, J.G.; Schmitz, M.D.; Ogg, G.M. (eds.). Geologic Time Scale 2020. Amsterdam: Elsevier. pp. 482–483. doi:10.1016/B978-0-12-824360-2.00016-4. ISBN 978-0-12-824360-2. S2CID 229513433.
- "International Chronostratigraphic Chart". International Commission on Stratigraphy. Retrieved 22 July 2020.
- Ogg, J. G.; Ogg, G.; Gradstein, F. M. (2016). A Concise Geologic Time Scale: 2016. Elsevier. p. 20. ISBN 978-0-444-63771-0.
- Korenaga, J (2021). "Was There Land on the Early Earth?". Life. 11 (11): 1142. doi:10.3390/life11111142. PMC 8623345. PMID 34833018.
- Cloud, Preston (1972). "A working model of the primitive Earth". American Journal of Science. 272 (6): 537–548. Bibcode:1972AmJS..272..537C. doi:10.2475/ajs.272.6.537.
- Bleeker, W. (2004). "10. Toward a "natural" Precambrian time scale". In Gradstein, Felix M.; Ogg, James G.; Smith, Alan G. (eds.). A Geologic Time Scale 2004. Cambridge, England, UK: Cambridge University Press. p. 145. ISBN 9780521786737.
- Oxford Dictionary, "Priscoan"
- Shaw, D.M. (1975). Early History of the Earth. Proceedings of the NATO Advanced Study Institute. Leicester: John Wiley. pp. 33–53. ISBN 0-471-01488-5.
- Jarvis, Gary T.; Campbell, Ian H. (December 1983). "Archean komatiites and geotherms: Solution to an apparent contradiction". Geophysical Research Letters. 10 (12): 1133–1136. Bibcode:1983GeoRL..10.1133J. doi:10.1029/GL010i012p01133.
- "The eons of Chaos and Hades" (PDF). Solid Earth. 26 January 2010.
- "Chart". International Commission on Stratigraphy. February 2022. Retrieved 8 July 2022.
- Borenstein, Seth (19 October 2015). "Hints of life on what was thought to be desolate early Earth". Excite. Yonkers, NY: Mindspark Interactive Network. Associated Press. Retrieved 2015-10-20.
- Bell, Elizabeth A.; Boehnike, Patrick; Harrison, T. Mark; et al. (19 October 2015). "Potentially biogenic carbon preserved in a 4.1 billion-year-old zircon". Proc. Natl. Acad. Sci. U.S.A. Washington, D.C.: National Academy of Sciences. 112 (47): 14518–21. Bibcode:2015PNAS..11214518B. doi:10.1073/pnas.1517557112. ISSN 1091-6490. PMC 4664351. PMID 26483481.
https://en.wikipedia.org/wiki/Hadean
Radiogenic heating
Radiogenic heating occurs as a result of the release of heat energy from radioactive decay[3] during the production of radiogenic nuclides. Along with heat from the Primordial Heat (resulting from planetary accretion), radiogenic heating occurring in the mantle and crust make up the two main sources of heat in the Earth's interior.[4] Most of the radiogenic heating in the Earth results from the decay of the daughter nuclei in the decay chains of uranium-238 and thorium-232, and potassium-40.[5]
See also
https://en.wikipedia.org/wiki/Radiogenic_nuclide#Radiogenic_heating
- In this table Gyr = gigayear = 109 year, Myr = megayear = 106 year, kyr = kiloyear = 103 year
Parent nuclide | Daughter nuclide | Decay constant (yr−1) | Half-life |
---|---|---|---|
190Pt | 186Os | 1.477 ×10−12 | 469.3 Gyr * |
147Sm | 143Nd | 6.54 ×10−12 | 106 Gyr |
87Rb | 87Sr | 1.402 ×10−11 | 49.44 Gyr |
187Re | 187Os | 1.666 ×10−11 | 41.6 Gyr |
176Lu | 176Hf | 1.867 ×10−11 | 37.1 Gyr |
232Th | 208Pb** | 4.9475 ×10−11 | 14.01 Gyr |
40K | 40Ar | 5.81 ×10−11 | 11.93 Gyr[2] |
238U | 206Pb** | 1.55125 ×10−10 | 4.468 Gyr |
40K | 40Ca | 4.962 ×10−10 | 1.397 Gyr |
235U | 207Pb** | 9.8485 ×10−10 | 0.7038 Gyr |
129I | 129Xe | 4.3 ×10−8 | 16 Myr |
10Be | 10B | 4.6 ×10−7 | 1.5 Myr |
26Al | 26Mg | 9.9 ×10−7 | 0.7 Myr |
36Cl | 36Ar/S | 2.24 ×10−6 | 310 kyr |
234U | 230Th | 2.826 ×10−6 | 245.25 kyr |
230Th | 226Ra | 9.1577 ×10−6 | 75.69 kyr |
231Pa | 227Ac | 2.116 ×10−5 | 32.76 kyr |
14C | 14N | 1.2097 ×10−4 | 5730 yr |
226Ra | 222Rn | 4.33 ×10−4 | 1600 yr |
https://en.wikipedia.org/wiki/Radiogenic_nuclide#Radiogenic_heating
An extinct radionuclide is a radionuclide that was formed by nucleosynthesis before the formation of the Solar System, about 4.6 billion years ago, but has since decayed to virtually zero abundance and is no longer detectable as a primordial nuclide. Extinct radionuclides were generated by various processes in the early Solar system, and became part of the composition of meteorites and protoplanets. All widely documented extinct radionuclides have half-lives shorter than 100 million years.[1]
Short-lived radioisotopes that are found in nature are continuously generated or replenished by natural processes, such as cosmic rays (cosmogenic nuclides), background radiation, or the decay chain or spontaneous fission of other radionuclides.
Short-lived isotopes that are not generated or replenished by natural processes are not found in nature, so they are known as extinct radionuclides. Their former existence is inferred from a superabundance of their stable or nearly stable decay products.
Examples of extinct radionuclides include iodine-129 (the first to be noted in 1960, inferred from excess xenon-129 concentrations in meteorites, in the xenon-iodine dating system), aluminium-26 (inferred from extra magnesium-26 found in meteorites), and iron-60.
The Solar System and Earth formed from primordial nuclides and extinct nuclides. Extinct nuclides have decayed away, but primordial nuclides still exist in their original state (undecayed). There are 251 stable primordial nuclides, and remainders of 35 primordial radionuclides that have very long half-lives.
https://en.wikipedia.org/wiki/Extinct_radionuclide
In nuclear science, the decay chain refers to a series of radioactive decays of different radioactive decay products as a sequential series of transformations. It is also known as a "radioactive cascade". Most radioisotopes do not decay directly to a stable state, but rather undergo a series of decays until eventually a stable isotope is reached.
https://en.wikipedia.org/wiki/Decay_chain
A geoneutrino is a neutrino or antineutrino emitted in decay of radionuclide naturally occurring in the Earth. Neutrinos, the lightest of the known subatomic particles, lack measurable electromagnetic properties and interact only via the weak nuclear force when ignoring gravity. Matter is virtually transparent to neutrinos and consequently they travel, unimpeded, at near light speed through the Earth from their point of emission. Collectively, geoneutrinos carry integrated information about the abundances of their radioactive sources inside the Earth. A major objective of the emerging field of neutrino geophysics involves extracting geologically useful information (e.g., abundances of individual geoneutrino-producing elements and their spatial distribution in Earth's interior) from geoneutrino measurements. Analysts from the Borexino collaboration have been able to get to 53 events of neutrinos originating from the interior of the Earth.[1]
https://en.wikipedia.org/wiki/Geoneutrino
List of extinct radionuclides
A partial list of radionuclides not found on Earth, but for which decay products are present:
Isotope | Halflife (Myr) | Daughter |
---|---|---|
Plutonium-244 | 80.8 | Thorium-232, fission products (especially xenon) |
Samarium-146 | 68.7 | Neodymium-142 (stable) |
Niobium-92 | 34.7 | Zirconium-92 (stable) |
Iodine-129 | 15.7 | Xenon-129 (stable) |
Curium-247 | 15.6 | Uranium-235 |
Lead-205 | 15.3 | Thallium-205 (stable) |
Hafnium-182 | 8.91 | Tungsten-182 (stable) |
Palladium-107 | 6.53 | Silver-107 (stable) |
Technetium-97 | 4.21 | Molybdenum-97 (stable) |
Technetium-98 | 4.2 | Ruthenium-98 (stable) |
Dysprosium-154 | 3.01 | Neodymium-142 (stable) |
Iron-60 | 2.62 | Nickel-60 (stable) |
Caesium-135 | 2.33 | Barium-135 (stable) |
Neptunium-237 | 2.144 | Bismuth-209 |
Gadolinium-150 | 1.798 | Neodymium-142 (stable) |
Zirconium-93 | 1.53 | Niobium-93 (stable) |
Aluminium-26 | 0.717 | Magnesium-26 (stable) |
Lanthanum-137 | 0.06 | Barium-137 (stable) |
Plutonium-244 and samarium-146 have half-lives long enough to still be present on Earth, but they have not been confirmed experimentally to be present.
Notable isotopes with shorter lives still being produced on Earth include:
- Manganese-53 and beryllium-10 are produced by cosmic ray spallation on dust in the upper atmosphere.
- Uranium-236 is produced in uranium ores by neutrons from other radioisotopes.
- Iodine-129 is produced from tellurium-130 by cosmic-ray muons and from cosmic ray spallation of stable xenon isotopes in the atmosphere.
Radioisotopes with half-lives shorter than one million years are also produced: for example, carbon-14 by cosmic ray production in the atmosphere (half-life 5730 years).
Use in geochronology
Despite the fact that the radioactive isotopes mentioned above are now effectively extinct, the record of their existence is found in their decay products and are very useful to geologists who wish to use them as geochronometers.[2] Their usefulness derives from a few factors such as the fact that their short half-lives provide high chronological resolution and the chemical mobility of various elements can date unique geological processes such as igneous fractionation and surface weathering. There are, however, hurdles to overcome when using extinct nuclides. The need for high-precision isotope ratio measurements is paramount as the extinct radionuclides contribute such a small fraction of the daughter isotopes. Compounding this problem is the increasing contribution that high-energy cosmic rays have on already minute amounts of daughter isotopes formed from the extinct nuclides. Distinguishing the source and abundance of these effects is critical to obtaining accurate ages from extinct nuclides. Additionally, more work needs to be done in determining a more precise half-life for some of these isotopes, such as 60Fe and 146Sm.[3]
See also
- Presolar grains
- Radiogenic nuclide, the dual concept
- Radiometric dating
- List of nuclides which includes a list of radionuclides in order by half-life
https://en.wikipedia.org/wiki/Extinct_radionuclide
Presolar grains are interstellar solid matter in the form of tiny solid grains that originated at a time before the Sun was formed. Presolar stardust grains formed within outflowing and cooling gases from earlier presolar stars.
The stellar nucleosynthesis that took place within each presolar star gives to each granule an isotopic composition unique to that parent star, which differs from the isotopic composition of our solar system's matter as well as from the galactic average. These isotopic signatures often fingerprint very specific astrophysical nuclear processes[1] that took place within the parent star and prove their presolar origin.[2][3]
History
In the 1960s, the noble gases neon[4] and xenon[5] were discovered to have unusual isotopic ratios in primitive meteorites; their origin and the type of matter that contained them was a mystery. These discoveries were made by vaporizing a bulk sample of a meteorite within a mass spectrometer, in order to count the relative abundance of the isotopes of the very small amount of noble gases trapped as inclusions. During the 1970s similar experiments discovered more components of trapped xenon isotopes.[6] Competing speculations about the origins of the xenon isotopic components were advanced, all within the existing paradigm that the variations were created by processes within an initially homogeneous solar gas cloud.
https://en.wikipedia.org/wiki/Presolar_grains
In meteorites
Presolar grains are the solid matter that was contained in the interstellar gas before the Sun formed. The stardust component can be identified in the laboratory by their abnormal isotopic abundances and consists of refractory minerals which survived the collapse of the solar nebula and the subsequent formation of planetesimals.[19]
To meteorite researchers, the term presolar grains has come to mean presolar grains found in meteorites, which consist overwhelmingly of stardust. Many other types of cosmic dust have not been detected in meteorites. Presolar stardust grains comprise only about 0.1 percent of the total mass of particulate matter found in meteorites. Such grains are isotopically-distinct material found in the fine-grained matrix of meteorites, such as primitive chondrites. [20] Their isotopic differences from the encasing meteorite require that they predate the Solar System. The crystallinity of those clusters ranges from micrometer-sized silicon carbide crystals (up to 1013 atoms), down to that of nanometer-sized diamond (about 1000 atoms), and unlayered graphene crystals of fewer than 100 atoms. The refractory grains achieved their mineral structures by condensing thermally within the slowly cooling expanding gases of supernovae and of red giant stars. [20]
https://en.wikipedia.org/wiki/Presolar_grains
Presolar grains consisting of the following minerals have so far been identified: diamond (C) nanometer-sized grains (~2.6 nanometres (1⁄10000000 in) diameter)[21] possibly formed by vapor deposition[22] graphite (C) particles and anions,[23] some with unlayered graphene cores[24] silicon carbide (SiC) submicrometer to micrometer sized grains. Presolar SiC occurs as single-polytype grains or polytype intergrowths. The atomic structures observed contain the two lowest order polytypes: hexagonal 2H and cubic 3C (with varying degrees of stacking fault disorder) as well as 1-dimensionally disordered SiC grains.[25] In comparison, SiC synthesized in terrestrial laboratories is known to form over a hundred polytypes. titanium carbide (TiC) and other carbides within C and SiC grains[26] silicon nitride (Si3N4) corundum (Al2O3)[27] spinel (MgAl2O4)[28] hibonite ((Ca,Ce)(Al,Ti,Mg)12O19)[29] titanium oxide (TiO2) silicate minerals (olivine and pyroxene) https://en.wikipedia.org/wiki/Presolar_grains Cosmic dust, also called extraterrestrial dust, star dust or space dust, is dust which exists in outer space, or has fallen on Earth.[1][2] Most cosmic dust particles measure between a few molecules and 0.1 mm (100 micrometers). Larger particles are called meteoroids. Cosmic dust can be further distinguished by its astronomical location: intergalactic dust, interstellar dust, interplanetary dust (such as in the zodiacal cloud) and circumplanetary dust (such as in a planetary ring). There are several methods to obtain space dust measurement. https://en.wikipedia.org/wiki/Cosmic_dust The Neandertal (/niˈændərˌtɑːl/, also US: /-ˌtɔːl/, German: [neˈʔandɐtʰaːl]; sometimes called "the Neander Valley" in English) is a small valley of the river Düssel in the German state of North Rhine-Westphalia, located about 12 km (7.5 mi) east of Düsseldorf, the capital city of North Rhine-Westphalia. The valley lies within the limits of the towns of Erkrath and Mettmann. In August 1856, the area became famous for the discovery of Neanderthal 1, one of the first specimens of Homo neanderthalensis to be found. https://en.wikipedia.org/wiki/Neandertal_(valley) Neanderthal Ancestors Homo habilis → Homo erectus (→ Homo antecessor)? → Homo heidelbergensis → Homo neanderthalensis Biology Anatomy Behaviour Genetics Admixture with H. sapiens Extinction Tool traditions Acheulean Mousterian Châtelperronian (controversial) Levallois technique Regional variants Gibraltar Neanderthals Southwest Asian Neanderthals Notable Specimens Archaic (430 ka) Miguelón Intermediate (250–130 ka) Saccopastore skulls Ehringsdorf 9 Altamura 1 Typical (130–50 ka) La Ferrassie 1 (site) La Chapelle-aux-Saints 1 (site) La Quina 5 and 18 Krijn Scladina Saccopastore 1 and 2 Altamura man Mt. Circeo 1 Krapina Neanderthal site Shanidar 1-4 Teshik-Tash 1 Kebara 2 (site) Tabun C1 Amud 1 and 7 (site) OR-1 In radiocarbon range (50–40 ka) Neanderthal 1 (type site) Spy 1 and 2 Engis 2 Sidrón remains Gibraltar 1 and 2 Saint-Césaire 1 Le Moustier Fontéchevade Mezmaiskaya 1 Fossils with claimed Neanderthal traits H. heidelbergensis Bontnewydd tooth Atapuerca Samu Azykhantrop Homo sapiens Peștera cu Oase Lagar Velho 1 Contemporary species Homo erectus Denisovan Homo floresiensis Homo naledi Homo sapiens Research Excavation sites Neanderthal Museum Neanderthal genome project (In Search of Lost Genomes) Popular culture The Quest for Fire (film) The Grisly Folk The Neanderthal Man The Inheritors The Ugly Little Boy Neanderthal Planet Dance of the Tiger Earth's Children (film) Neanderthal The Neanderthal Parallax Documentaries Prehistoric Autopsy https://en.wikipedia.org/wiki/Neanderthal https://en.wikipedia.org/wiki/Interbreeding_between_archaic_and_modern_humans#Neanderthals Neanderthals became extinct around 40,000 years ago. This timing, based on research published in Nature in 2014, is much earlier than previous estimates, and derives from improved radiocarbon dating methods analyzing 40 sites from Spain to Russia.[1] Evidence for continued Neanderthal presence in the Iberian Peninsula 37,000 years ago was published in 2017.[2] Various hypotheses on the causes of Neanderthal extinction implicate: violence; parasites and pathogens; competitive replacement;[3] extinction by interbreeding with early modern human populations;[4] natural catastrophes; climate change; inbreeding depression.[5][6] It seems unlikely that any single one of these hypotheses is sufficient on its own; rather, multiple factors probably contributed to the demise of an already low population. https://en.wikipedia.org/wiki/Neanderthal_extinction Homo habilis ("handy man") is an extinct species of archaic human from the Early Pleistocene of East and South Africa about 2.31 million years ago to 1.65 million years ago (mya). Upon species description in 1964, H. habilis was highly contested, with many researchers recommending it be synonymised with Australopithecus africanus, the only other early hominin known at the time, but H. habilis received more recognition as time went on and more relevant discoveries were made. By the 1980s, H. habilis was proposed to have been a human ancestor, directly evolving into Homo erectus which directly led to modern humans. This viewpoint is now debated. Several specimens with insecure species identification were assigned to H. habilis, leading to arguments for splitting, namely into "H. rudolfensis" and "H. gautengensis" of which only the former has received wide support. https://en.wikipedia.org/wiki/Homo_habilis Polygyny (/pəˈlɪdʒɪni/; from Neoclassical Greek πολυγυνία (polugunía); from Ancient Greek πολύ (polú) 'many', and γυνή (gunḗ) 'woman, wife')[1] is the most common and accepted form of polygamy around the world, entailing the marriage of a man with several women. Contents 1 Incidence 2 Cause and explanation 2.1 Augmenting division of labor 2.2 Desire for progeny 2.3 Economic burden 2.4 Libido 2.5 Enslavement of women https://en.wikipedia.org/wiki/Polygyny Types of marriages Legal scenarios Civil Covenant Customary Nikah 'urfi Plaçage Fleet Monogamy Serial monogamy Marriage Remarriage Same-sex Temporary Hollywood Nikah mut'ah Wedlease Voidable Putative Void Religious Jewish Christian Catholic Josephite Eastern Orthodox Mormon Quaker Clerical marriage Islamic Marriage in Islam Misyar Nikah mut'ah Nikah 'urfi Levirate Yibbum Natural Scientology Self-uniting Widow conservation Hindu Vivaaha Brahma Daiva Arsha Prajapatya Gandharva Asura Rakshasa Paishacha Ayyavazhi Vedic Yogic Age Marriageable age Child Teen Arranged Sister exchange Flash Forced Abduction Raptio Heqin Miai Shotgun Tongyangxi Ceremonial Betrothal Handfasting Mystery of Crowning Wedding Las Vegas Proxy Circumstantial basis Death Posthumous France Germany Chinese ghost marriage Widow inheritance Ghost marriage in South Sudan Levirate Yibbum Financial Flash Hypergamy Naked Peer Tongyangxi Trial Convenience Lavender Sham Green card Marriage allowance Predatory Other Exchange Watta satta Heqin Love Sologamy Mixed-orientation Sororate De facto Common-law Plaçage Endogamy Consanguine Avunculate Cousin Middle Eastern Same-surname Homogamy Royal intermarriage Exogamy Human–animal Hypergamy Inter-caste Interethnic International Interracial Interfaith Morganatic Non-monogamous Group Line Open Plural Bigamy Polygamy Polyandry Polygyny Sexless Mariage blanc Other Boston Concubinage Dishu system Mail-order bride Marriage in pre-Islamic Arabia Walking https://en.wikipedia.org/wiki/Polygyny Sister exchange is a type of marriage agreement where two sets of siblings marry each other. In order to get married, a man needs to persuade his sister to marry the bride's brother. It is practised as a primary method of organising marriages in 3% of the world's societies: in some tribes from Australia, Melanesia, Amazonia and Sub-Saharan Africa; and can replace other methods in 1.4% of the societies.[1] Researchers disagree about the reasoning behind sister exchange but most believe that it is some type of reciprocity. Several anthropologists and sociologists expressed objections to the term "sister exchange" believing that it is not accurately describing the actual arrangement.[2][3][4] Despite earlier claims of its simplicity, sister exchange is a complex arrangement that involves many family members and not simply the four people who are getting married.[5][6] https://en.wikipedia.org/wiki/Sister_exchange The coefficient of inbreeding of an individual is the probability that two alleles at any locus in an individual are identical by descent from the common ancestor(s) of the two parents.[1] The coefficient of inbreeding is: The probability that two alleles at a given locus are identical by descent.[2] The coefficient of relationship is: The proportion of genes that are held in common by two individuals as a result of direct or collateral relationship.[2] https://en.wikipedia.org/wiki/Coefficient_of_inbreeding This article needs additional citations for verification. Please help improve this article by adding citations to reliable sources. Unsourced material may be challenged and removed. Find sources: "Purebred" – news · newspapers · books · scholar · JSTOR (September 2007) (Learn how and when to remove this template message) Purebreds are "cultivated varieties" of an animal species achieved through the process of selective breeding. When the lineage of a purebred animal is recorded, that animal is said to be "pedigreed". Purebreds breed true-to-type which means the progeny of like-to-like purebred parents will carry the same phenotype, or observable characteristics of the parents. A group of purebreds is called a pure-breeding line or strain. https://en.wikipedia.org/wiki/Purebred Because pure-breeding creates a limited gene pool, purebred animal breeds are also susceptible to a wide range of congenital health problems.[2] This problem is especially prevalent in competitive dog breeding and dog show circles due to the singular emphasis on aesthetics rather than health or function. Such problems also occur within certain segments of the horse industry for similar reasons. The problem is further compounded when breeders practice inbreeding.[3] The opposite effect to that of the restricted gene pool caused by pure-breeding is known as hybrid vigor, which generally results in healthier animals.[2] https://en.wikipedia.org/wiki/Purebred Jump to navigation Jump to search A true-breeding organism, sometimes also called a purebred (biology slang: pure line or true-breeding line), is an organism that always passes down certain phenotypic traits (i.e. physically expressed traits) to its offspring of many generations. An organism is referred to as true breeding for each trait to which this applies, and the term "true-breeding" is also used to describe individual genetic traits.[1] In Mendelian genetics, this means that an organism must be homozygous for every trait for which it is considered true breeding; that is, the pairs of alleles that express a given trait are the same. In a purebred strain or breed, the goal is that the organism will "breed true" for the breed-relevant traits.[citation needed] Apomixis and parthenogenesis, types of asexual reproduction, also result in true breeding, although the organisms are usually not homozygous.[2] https://en.wikipedia.org/wiki/True-breeding_organism Inbreeding is the production of offspring from the mating or breeding of individuals or organisms that are closely related genetically.[2] By analogy, the term is used in human reproduction, but more commonly refers to the genetic disorders and other consequences that may arise from expression of deleterious or recessive traits resulting from incestuous sexual relationships and consanguinity. Animals avoid incest only rarely.[3] https://en.wikipedia.org/wiki/Inbreeding The Siamese cat (Thai: แมวไทย, Maeo Thai; แมวสยาม, Maeo Seeaam) is one of the first distinctly recognized breeds of Asian cat. Derived from the Wichianmat landrace, one of several varieties of cat native to Thailand (formerly known as Siam), the original Siamese became one of the most popular breeds in Europe and North America in the 19th century. The carefully refined, more extreme-featured, modern-style Siamese is characterized by blue almond-shaped eyes; a triangular head shape; large ears; an elongated, slender, and muscular body; and various forms of point colouration. Other than colouration, the modern-style Siamese bears little resemblance to the original stock, and the more moderate, traditional, or "old-style" Siamese, with a much rounder head and body, has been re-established by multiple registries as the Thai cat. The International Cat Association describes the modern Siamese as affectionate, social, intelligent, and playful into adulthood, often enjoying a game of fetch.[1] Siamese tend to seek human interaction and also like companionship from other cats. https://en.wikipedia.org/wiki/Siamese_cat The word "pedigree" appeared in the English language in 1410 as "pee de Grewe", "pedegrewe" or "pedegru", each of those words being borrowed to the Middle French "pié de grue", meaning "crane foot". This comes from a visual analogy between the trace of the bird's foot and the three lines used in the English official registers to show the ramifications of a genealogical tree.[4] https://en.wikipedia.org/wiki/Purebred Certain horse breeds, such as the Andalusian horse and the Arabian horse, are claimed by aficionados of the respective breeds to be ancient, near-pure descendants from an ancient wild prototype, though mapping of the horse genome as well as the mtDNA and y-DNA of various breeds has largely disproved such claims. https://en.wikipedia.org/wiki/Purebred https://en.wikipedia.org/wiki/Backcrossing https://en.wikipedia.org/wiki/Purebred https://en.wikipedia.org/wiki/Mutation_breeding https://en.wikipedia.org/wiki/Preservation_breeding https://en.wikipedia.org/wiki/Rare_breed
https://en.wikipedia.org/wiki/Rare_breed
https://en.wikipedia.org/wiki/Dog_coat_genetics
https://en.wikipedia.org/wiki/Albinism
https://en.wikipedia.org/wiki/Cream_gene
https://en.wikipedia.org/wiki/White_horse
https://en.wikipedia.org/wiki/Yeast
https://en.wikipedia.org/wiki/Dominant_white
https://en.wikipedia.org/wiki/Silver_dapple_gene
https://en.wikipedia.org/wiki/Equine_coat_color_genetics
https://en.wikipedia.org/wiki/White_horse#Why_cream_is_not_albino
https://en.wikipedia.org/wiki/Cat_coat_genetics
https://en.wikipedia.org/wiki/Tabby_cat
https://en.wikipedia.org/wiki/Pearl_gene
https://en.wikipedia.org/wiki/Point_coloration
https://en.wikipedia.org/wiki/Gray_horse
https://en.wikipedia.org/wiki/Ayam_Cemani
https://en.wikipedia.org/wiki/Silkie
https://en.wikipedia.org/wiki/Dilution_gene
In plants, albinism is characterised by partial or complete loss of chlorophyll pigments and incomplete differentiation of chloroplast membranes. Albinism in plants interferes with photosynthesis, which can reduce survivability.[9] Some plant variations may have white flowers or other parts. However, these plants are not totally devoid of chlorophyll. Terms associated with this phenomenon are "hypochromia" and "albiflora".[10]

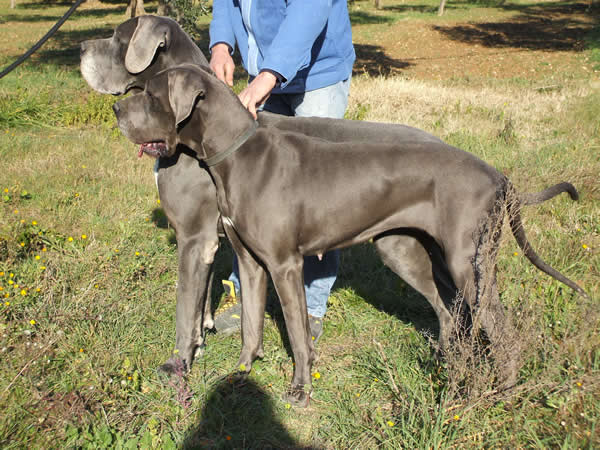




- Acne
- Asthma
- Calicivirus
- Congenital sensorineural deafness
- Feline corneal sequestrum
- Flea
- Coccidia
- Heartworm
- Hepatic lipidosis
- Hypertrophic cardiomyopathy
- Immunodeficiency virus
- Infectious peritonitis
- Leukemia virus
- Lower urinary tract disease
- Panleukopenia
- Giardiasis
- Polydactyly
- Rabies
- Ringworm
- Tritrichomonas foetus
- Roundworm
- Skin disorders
- Tick
- Toxoplasmosis
- Viral rhinotracheitis
https://en.wikipedia.org/wiki/Colorpoint_Shorthair
- Chinese mountain cat (F. bieti)
- Domestic cat (F. catus)
- Jungle cat (F. chaus)
- African wildcat (F. lybica)
- Sand cat (F. margarita)
- Black-footed cat (F. nigripes)
- European wildcat (F. silvestris)
https://en.wikipedia.org/wiki/Prionailurus
Southern tiger cat | |
---|---|
Scientific classification ![]() | |
Kingdom: | Animalia |
Phylum: | Chordata |
Class: | Mammalia |
Order: | Carnivora |
Suborder: | Feliformia |
Family: | Felidae |
Subfamily: | Felinae |
Genus: | Leopardus |
Species: | L. guttulus
|
Binomial name | |
Leopardus guttulus (Hensel, 1872)
| |
![]() | |
Distribution of the southern tigrina, 2016[1] |
https://en.wikipedia.org/wiki/Leopardus_guttulus
No comments:
Post a Comment