An ultra-short period (USP) planet is a type of exoplanet with orbital period less than one day.[1] At this short distance, tidal interactions lead to relatively rapid orbital and spin evolution. Therefore when there is a USP planet around a mature main-sequence star it is most likely that the planet has a circular orbit and is tidally locked.[1] There are not many USP planets with sizes exceeding 2 Earth radii.[1] About one out of 200 Sun-like stars (G dwarfs) has an ultra-short-period planet. There is a strong dependence of the occurrence rate on the mass of the host star. The occurrence rate falls from (1.1 ± 0.4)% for M dwarfs to (0.15 ± 0.05)% for F dwarfs.[1] Mostly the USP planets seem consistent with an Earth-like composition of 70% rock and 30% iron, but K2-229b has a higher density suggesting a more massive iron core. WASP-47e and 55 Cnc e have a lower density and are compatible with pure rock, or a rocky-iron body surrounded by a layer of water (or other volatiles).[1]
A difference between hot Jupiters and terrestrial USP planets is the proximity of planetary companions. Hot Jupiters are rarely found with other planets within a factor of 2–3 in orbital period or distance. In contrast, terrestrial USP planets almost always have longer-period planetary companions.[1] The period ratio between adjacent planets tends to be larger if one of them is a USP planet suggesting the USP planet has undergone tidal orbital decay which may still be ongoing.[1] USP planets also tend to have higher mutual inclinations with adjacent planets than for pairs of planets in wider orbits, suggesting that USP planets have experienced inclination excitation in addition to orbital decay.[1]
There are several known giant planets with a period shorter than one day. Their occurrence must be lower by at least an order of magnitude than that of terrestrial USP planets.[1]
It had been proposed that USP planets were the rocky cores of evaporated hot Jupiters, however the metallicity of the host stars of USP planets is lower than that of hot Jupiters' stars so it seems more likely that USP planets are the cores of evaporated gas dwarfs.[1]
https://en.wikipedia.org/wiki/Ultra-short_period_planet
An F-type main-sequence star (F V) is a main-sequence, hydrogen-fusing star of spectral type F and luminosity class V. These stars have from 1.0 to 1.4 times the mass of the Sun and surface temperaturesbetween 6,000 and 7,600 K.[2]Tables VII and VIII. This temperature range gives the F-type stars a yellow-white hue. Because a main-sequence star is referred to as a dwarf star, this class of star may also be termed a yellow-white dwarf (not to be confused with white dwarfs, a possible final stage of stellar evolution). Famous examples include Procyon A, Gamma Virginis A and B,[3] and KIC 8462852.
https://en.wikipedia.org/wiki/F-type_main-sequence_star#Planets
A B-type subdwarf (sdB) is a kind of subdwarf star with spectral type B. They differ from the typical subdwarf by being much hotter and brighter.[2] They are situated at the "extreme horizontal branch" of the Hertzsprung–Russell diagram. Masses of these stars are around 0.5 solar masses, and they contain only about 1% hydrogen, with the rest being helium. Their radius is from 0.15 to 0.25 solar radii, and their temperature is from 20,000 to 40,000K.
These stars represent a late stage in the evolution of some stars, caused when a red giant star loses its outer hydrogen layers before the core begins to fuse helium. The reasons why this premature mass loss occurs are unclear, but the interaction of stars in a binary star system is thought to be one of the main mechanisms. Single subdwarfs may be the result of a merger of two white dwarfs. The sdB stars are expected to become white dwarfs without going through any more giant stages.
Subdwarf B stars, being more luminous than white dwarfs, are a significant component in the hot star population of old stellar systems, such as globular clusters, spiral galaxy bulges and elliptical galaxies.[3]They are prominent on ultraviolet images. The hot subdwarfs are proposed to be the cause of the UV upturn in the light output of elliptical galaxies.[2]
An A-type main-sequence star (A V) or A dwarf star is a main-sequence (hydrogen-burning) star of spectral type A and luminosity class V (five). These stars have spectra which are defined by strong hydrogen Balmer absorption lines.[1][2] They measure between 1.4 and 2.1 solar masses (M☉) and have surface temperaturesbetween 7600 and 10,000 K.[3] Bright and nearby examples are Altair (A7 V), Sirius A (A1 V), and Vega (A0 V).[4] A-type stars don't have a convective zone and thus aren't expected to harbor a magnetic dynamo. As a consequence, because they don't have strong stellar winds they lack a means to generate X-ray emission.[5]
In July 2019, astronomers reported finding an A-type star, S5-HVS1, traveling 1,755 km/s (3,930,000 mph), faster than any other star detected so far. The star is in the Grus (or Crane) constellation in the southern sky, and about 29,000 light-years from Earth, and may have been ejected out of the Milky Way galaxy after interacting with Sagittarius A*, the supermassive black hole at the center of the galaxy.[6][7][8][9][10]
https://en.wikipedia.org/wiki/A-type_main-sequence_star#Planets
A B-type main-sequence star (B V) is a main-sequence (hydrogen-burning) star of spectral type B and luminosity class V. These stars have from 2 to 16 times the mass of the Sun and surface temperatures between 10,000 and 30,000 K.[2] B-type stars are extremely luminous and blue. Their spectra have neutral helium, which are most prominent at the B2 subclass, and moderate hydrogen lines. Examples include Regulusand Algol A.[3]
This class of stars was introduced with the Harvard sequence of stellar spectra and published in the Revised Harvard photometrycatalogue. The definition of type B-type stars was the presence of non-ionized helium lines with the absence of singly ionized helium in the blue-violet portion of the spectrum. All of the spectral classes, including the B type, were subdivided with a numerical suffix that indicated the degree to which they approached the next classification. Thus B2 is 1/5 of the way from type B (or B0) to type A.[4][5]
Later, however, more refined spectra showed lines of ionized helium for stars of type B0. Likewise, A0 stars also show weak lines of non-ionized helium. Subsequent catalogues of stellar spectra classified the stars based on the strengths of absorption lines at specific frequencies, or by comparing the strengths of different lines. Thus, in the MK Classification system, the spectral class B0 has the line at wavelength 439 nm being stronger than the line at 420 nm.[6] The Balmer series of hydrogen lines grows stronger through the B class, then peak at type A2. The lines of ionized silicon are used to determine the sub-class of the B-type stars, while magnesium lines are used to distinguish between the temperature classes.[5]
Type-B stars don't have a corona and lack a convection zone in their outer atmosphere. They have a higher mass loss rate than smaller stars such as the Sun, and their stellar wind has velocities of about 3,000 km/s.[7] The energy generation in main-sequence B-type stars comes from the CNO cycle of thermonuclear fusion. Because the CNO cycle is very temperature sensitive, the energy generation is heavily concentrated at the center of the star, which results in a convection zone about the core. This results in a steady mixing of the hydrogen fuel with the helium byproduct of the nuclear fusion.[8]Many B-type stars have a rapid rate of rotation, with an equatorial rotation velocity of about 200 km/s.[9]
Spectral Type | Radius R☉ | Mass M☉ | Teff (K) | log g |
---|---|---|---|---|
B0V | 10 | 17 | 30,000 | 4 |
B1V | 6.42 | 13.21 | 25,400 | 3.9 |
B2V | 5.33 | 9.11 | 20,800 | 3.9 |
B3V | 4.8 | 7.6 | 18,800 | 4 |
B5V | 3.9 | 5.9 | 15,200 | 4 |
B6V | 3.56 | 5.17 | 13,800 | 4 |
B7V | 3.28 | 4.45 | 12,400 | 4.1 |
B8V | 3 | 3.8 | 11,400 | 4.1 |
B9V | 2.7 | 3.29 | 10,600 | 4.1 |
https://en.wikipedia.org/wiki/B-type_main-sequence_star#Planets
F-Type Star
Some studies show that there is a possibility that life could also develop on planets that orbit an F-type star.[10] It is estimated that the habitable zone of a relatively hot F0 star would extend from about 2.0 AU to 3.7 AU and between 1.1 and 2.2 AU for a relatively cool F8 star.[10] However, relative to a G-type star the main problems for a hypothetical lifeform in this particular scenario would be the more intense light and the shorter stellar lifespan of the home star.[10]
F-type stars are known to emit much higher energy forms of light, such as UV radiation, which in the long term can have a profoundly negative effect on DNA molecules.[10] Studies have shown that, for a hypothetical planet positioned at an equivalent habitable distance from an F-type star as the Earth is from the Sun (this is further away from the F-type star, inside the habitable zone), and with a similar atmosphere, life on its surface would receive about 2.5 to 7.1 times more damage from UV light compared to that on Earth.[11] Thus, for its native lifeforms to survive, the hypothetical planet would need to have sufficient atmospheric shielding, such as an ozone layer in the upper atmosphere.[10] Without a robust ozone layer, life could theoretically develop on the planet's surface, but it would most likely be confined to underwater or underground regions.[10]
https://en.wikipedia.org/wiki/F-type_main-sequence_star#Planets
SUN
The Sun is the star at the center of the Solar System. It is a nearly perfect sphere of hot plasma,[18][19]heated to incandescence by nuclear fusion reactions in its core, radiating the energy mainly as visible light, ultraviolet light, and infrared radiation. It is by far the most important source of energy for life on Earth. Its diameter is about 1.39 million kilometres (864,000 miles), or 109 times that of Earth. Its mass is about 330,000 times that of Earth; it accounts for about 99.86% of the total mass of the Solar System.[20]Roughly three quarters of the Sun's mass consists of hydrogen (~73%); the rest is mostly helium (~25%), with much smaller quantities of heavier elements, including oxygen, carbon, neon and iron.[21]
The Sun is a G-type main-sequence star (G2V) based on its spectral class. As such, it is informally and not completely accurately referred to as a yellow dwarf (its light is closer to white than yellow). It formed approximately 4.6 billion[a][14][22] years ago from the gravitational collapse of matter within a region of a large molecular cloud. Most of this matter gathered in the center, whereas the rest flattened into an orbiting disk that became the Solar System. The central mass became so hot and dense that it eventually initiated nuclear fusion in its core. It is thought that almost all stars form by this process.
The Sun's core fuses about 600 million tons of hydrogen into helium every second, converting 4 million tons of matter into energy every second as a result. This energy, which can take between 10,000 and 170,000 years to escape the core, is the source of the Sun's light and heat. When hydrogen fusion in its core has diminished to the point at which the Sun is no longer in hydrostatic equilibrium, its core will undergo a marked increase in density and temperature while its outer layers expand, eventually transforming the Sun into a red giant. It is calculated that the Sun will become sufficiently large to engulf the current orbits of Mercury and Venus, and render Earth uninhabitable – but not for about five billion years. After this, it will shed its outer layers and become a dense type of cooling star known as a white dwarf, and no longer produce energy by fusion, but still glow and give off heat from its previous fusion.
The enormous effect of the Sun on Earth has been recognized since prehistoric times. The Sun was thought of by some cultures as a deity. The synodic rotation of Earth and its orbit around the Sun are the basis of solar calendars, one of which is the Gregorian calendar, the predominant calendar in use today.
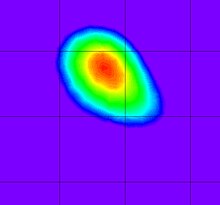
Through most of the Sun's life, energy has been produced by nuclear fusion in the core region through a series of nuclear reactions called the p–p (proton–proton) chain; this process converts hydrogen into helium.[71] Only 0.8% of the energy generated in the Sun comes from another sequence of fusion reactions called the CNO cycle, though this proportion is expected to increase as the Sun becomes older.[72][73]
The core is the only region in the Sun that produces an appreciable amount of thermal energy through fusion; 99% of the power is generated within 24% of the Sun's radius, and by 30% of the radius, fusion has stopped nearly entirely. The remainder of the Sun is heated by this energy as it is transferred outwards through many successive layers, finally to the solar photosphere where it escapes into space through radiation (photons) or advection (massive particles).[53][74]
The proton–proton chain occurs around 9.2×1037 times each second in the core, converting about 3.7×1038 protons into alpha particles (helium nuclei) every second (out of a total of ~8.9×1056 free protons in the Sun), or about 6.2×1011 kg/s.[53] Fusing four free protons (hydrogen nuclei) into a single alpha particle (helium nucleus) releases around 0.7% of the fused mass as energy,[75] so the Sun releases energy at the mass–energy conversion rate of 4.26 million metric tons per second (which requires 600 metric megatons of hydrogen [76]), for 384.6 yottawatts (3.846×1026 W),[5] or 9.192×1010 megatons of TNT per second. The large power output of the Sun is mainly due to the huge size and density of its core (compared to Earth and objects on Earth), with only a fairly small amount of power being generated per cubic metre. Theoretical models of the Sun's interior indicate a maximum power density, or energy production, of approximately 276.5 watts per cubic metre at the center of the core,[77] which is about the same power density inside a compost pile.[78][f]
The fusion rate in the core is in a self-correcting equilibrium: a slightly higher rate of fusion would cause the core to heat up more and expand slightly against the weight of the outer layers, reducing the density and hence the fusion rate and correcting the perturbation; and a slightly lower rate would cause the core to cool and shrink slightly, increasing the density and increasing the fusion rate and again reverting it to its present rate.[79][80]
- Core – the innermost 20–25% of the Sun's radius, where temperature and pressure are sufficient for nuclear fusion to occur. Hydrogen fuses into helium (which cannot itself be fused at this point in the Sun's life). The fusion process releases energy, and the core gradually becomes enriched in helium.
- Radiative zone – Convection cannot occur until much nearer the surface of the Sun. Therefore, between about 20–25% of the radius, and 70% of the radius, there is a "radiative zone" in which energy transfer occurs by means of radiation (photons) rather than by convection.
- Tachocline – the boundary region between the radiative and convective zones.
- Convective zone – Between about 70% of the Sun's radius and a point close to the visible surface, the Sun is cool and diffuse enough for convection to occur, and this becomes the primary means of outward heat transfer, similar to weather cells which form in the earth's atmosphere.
- Photosphere – the deepest part of the Sun which we can directly observe with visible light.
- Atmosphere – a gaseous "halo" surrounding the Sun, comprising the chromosphere, solar transition region, corona and heliosphere. These can be seen when the main part of the Sun is hidden, for example, during a solar eclipse.
A corona (Latin for 'crown', in turn derived from Ancient Greek κορώνη, korṓnē, 'garland, wreath') is an aura of plasma that surrounds the Sun and other stars. The Sun's corona extends millions of kilometres into outer space and is most easily seen during a total solar eclipse, but it is also observable with a coronagraph. Spectroscopymeasurements indicate strong ionization in the corona and a plasma temperature in excess of 1000000 kelvin,[1] much hotter than the surface of the Sun.
Light from the corona comes from three main sources, from the same volume of space:
- The K-corona (K for kontinuierlich, "continuous" in German) is created by sunlight scattering off free electrons; Doppler broadening of the reflected photospheric absorption lines spreads them so greatly as to completely obscure them, giving the spectral appearance of a continuum with no absorption lines.
- The F-corona (F for Fraunhofer) is created by sunlight bouncing off dust particles, and is observable because its light contains the Fraunhofer absorption lines that are seen in raw sunlight; the F-corona extends to very high elongation angles from the Sun, where it is called the zodiacal light.
- The E-corona (E for emission) is due to spectral emission lines produced by ions that are present in the coronal plasma; it may be observed in broad or forbidden or hot spectral emission lines and is the main source of information about the corona's composition.[2]
In atomic physics, hyperfine structure is defined by small shifts in otherwise degenerate energy levels and the resulting splittings in those energy levelsof atoms, molecules, and ions, due to interaction between the nucleus and electron clouds.
In atoms, hyperfine structure arises from the energy of the nuclear magnetic dipole moment interacting with the magnetic field generated by the electrons and the energy of the nuclear electric quadrupole moment in the electric field gradient due to the distribution of charge within the atom. Molecular hyperfine structure is generally dominated by these two effects, but also includes the energy associated with the interaction between the magnetic moments associated with different magnetic nuclei in a molecule, as well as between the nuclear magnetic moments and the magnetic field generated by the rotation of the molecule.
Hyperfine structure contrasts with fine structure, which results from the interaction between the magnetic moments associated with electron spin and the electrons' orbital angular momentum. Hyperfine structure, with energy shifts typically orders of magnitudes smaller than those of a fine-structure shift, results from the interactions of the nucleus (or nuclei, in molecules) with internally generated electric and magnetic fields.
https://en.wikipedia.org/wiki/Hyperfine_structure
The cosmic microwave background (CMB, CMBR), in Big Bang cosmology, is electromagnetic radiationwhich is a remnant from an early stage of the universe, also known as "relic radiation".[1] The CMB is faint cosmic background radiation filling all space. It is an important source of data on the early universe because it is the oldest electromagnetic radiation in the universe, dating to the epoch of recombination. With a traditional optical telescope, the space between stars and galaxies (the background) is completely dark. However, a sufficiently sensitive radio telescope shows a faint background noise, or glow, almost isotropic, that is not associated with any star, galaxy, or other object. This glow is strongest in the microwave region of the radio spectrum. The accidental discovery of the CMB in 1965 by American radio astronomers Arno Penzias and Robert Wilson[2][3] was the culmination of work initiated in the 1940s, and earned the discoverers the 1978 Nobel Prize in Physics.
CMB is landmark evidence of the Big Bang origin of the universe. When the universe was young, before the formation of stars and planets, it was denser, much hotter, and filled with an opaque fog of hydrogen plasma. As the universe expanded, both the plasma and the radiation filling it grew cooler. When the temperature had dropped enough, protons and electrons combined to form neutral hydrogen atoms. Unlike the plasma, these newly conceived atoms could not scatter the thermal radiation by Thomson scattering, and so the universe became transparent.[4] Cosmologists refer to the time period when neutral atoms first formed as the recombination epoch, and the event shortly afterwards when photons started to travel freely through space is referred to as photon decoupling. The photons that existed at the time of photon decoupling have been propagating ever since, though growing fainter and less energetic, since the expansion of space causes their wavelength to increase over time (and wavelength is inversely proportional to energy according to Planck's relation). This is the source of the alternative term relic radiation. The surface of last scattering refers to the set of points in space at the right distance from us so that we are now receiving photons originally emitted from those points at the time of photon decoupling.
Photons and neutrinos
High-energy gamma ray photons initially released with fusion reactions in the core are almost immediately absorbed by the solar plasma of the radiative zone, usually after traveling only a few millimeters. Re-emission happens in a random direction and usually at slightly lower energy. With this sequence of emissions and absorptions, it takes a long time for radiation to reach the Sun's surface. Estimates of the photon travel time range between 10,000 and 170,000 years.[102] In contrast, it takes only 2.3 seconds for the neutrinos, which account for about 2% of the total energy production of the Sun, to reach the surface. Because energy transport in the Sun is a process that involves photons in thermodynamic equilibrium with matter, the time scale of energy transport in the Sun is longer, on the order of 30,000,000 years. This is the time it would take the Sun to return to a stable state if the rate of energy generation in its core were suddenly changed.[103]
Neutrinos are also released by the fusion reactions in the core, but, unlike photons, they rarely interact with matter, so almost all are able to escape the Sun immediately. For many years measurements of the number of neutrinos produced in the Sun were lower than theories predicted by a factor of 3. This discrepancy was resolved in 2001 through the discovery of the effects of neutrino oscillation: the Sun emits the number of neutrinos predicted by the theory, but neutrino detectors were missing 2⁄3 of them because the neutrinos had changed flavor by the time they were detected.[104]
The solar magnetic field extends well beyond the Sun itself. The electrically conducting solar wind plasma carries the Sun's magnetic field into space, forming what is called the interplanetary magnetic field.[94] In an approximation known as ideal magnetohydrodynamics, plasma particles only move along the magnetic field lines. As a result, the outward-flowing solar wind stretches the interplanetary magnetic field outward, forcing it into a roughly radial structure. For a simple dipolar solar magnetic field, with opposite hemispherical polarities on either side of the solar magnetic equator, a thin current sheet is formed in the solar wind.[94] At great distances, the rotation of the Sun twists the dipolar magnetic field and corresponding current sheet into an Archimedean spiral structure called the Parker spiral.[94] The interplanetary magnetic field is much stronger than the dipole component of the solar magnetic field. The Sun's dipole magnetic field of 50–400 μT (at the photosphere) reduces with the inverse-cube of the distance, leading to a predicted magnetic field of 0.1 nT at the distance of Earth. However, according to spacecraft observations the interplanetary field at Earth's location is around 5 nT, about a hundred times greater.[114] The difference is due to magnetic fields generated by electrical currents in the plasma surrounding the Sun.
https://en.wikipedia.org/wiki/Sun
A coronal mass ejection (CME) is a significant release of plasma and accompanying magnetic field from the solar corona. They often follow solar flares and are normally present during a solar prominence eruption. The plasma is released into the solar wind, and can be observed in coronagraph imagery.[1][2][3]
Coronal mass ejections are often associated with other forms of solar activity, but a broadly accepted theoretical understanding of these relationships has not been established. CMEs most often originate from active regions on the Sun's surface, such as groupings of sunspots associated with frequent flares. Near solar maxima, the Sun produces about three CMEs every day, whereas near solar minima, there is about one CME every five days.[4]
The largest recorded geomagnetic perturbation, resulting presumably from a CME hitting the Earth's magnetosphere, was the solar storm of 1859 (the Carrington Event), which took down parts of the recently created US telegraph network, starting fires and shocking some telegraph operators.[5]
Heliophysics |
---|
Phenomena |
https://en.wikipedia.org/wiki/Coronal_mass_ejection
Magnetohydrodynamics (MHD; also magneto-fluid dynamics or hydromagnetics) is the study of the magnetic properties and behaviour of electrically conducting fluids. Examples of such magnetofluids include plasmas, liquid metals, salt water, and electrolytes. The word "magnetohydrodynamics" is derived from magneto- meaning magnetic field, hydro- meaning water, and dynamics meaning movement. The field of MHD was initiated by Hannes Alfvén,[1] for which he received the Nobel Prize in Physics in 1970.
The fundamental concept behind MHD is that magnetic fields can induce currents in a moving conductive fluid, which in turn polarizes the fluid and reciprocally changes the magnetic field itself. The set of equations that describe MHD are a combination of the Navier–Stokes equations of fluid dynamics and Maxwell’s equations of electromagnetism. These differential equations must be solved simultaneously, either analytically or numerically.
https://en.wikipedia.org/wiki/Magnetohydrodynamics
In December 2019, a new type of solar magnetic explosion was observed, known as forced magnetic reconnection. Previously, in a process called spontaneous magnetic reconnection, it was observed that the solar magnetic field lines diverge explosively and then converge again instantaneously. Forced Magnetic Reconnection was similar, but it was triggered by an explosion in the corona.[116]
Long-term change
Long-term secular change in sunspot number is thought, by some scientists, to be correlated with long-term change in solar irradiance,[117] which, in turn, might influence Earth's long-term climate.[118] For example, in the 17th century, the solar cycle appeared to have stopped entirely for several decades; few sunspots were observed during a period known as the Maunder minimum. This coincided in time with the era of the Little Ice Age, when Europe experienced unusually cold temperatures.[119] Earlier extended minima have been discovered through analysis of tree rings and appear to have coincided with lower-than-average global temperatures.[120]
A recent theory claims that there are magnetic instabilities in the core of the Sun that cause fluctuations with periods of either 41,000 or 100,000 years. These could provide a better explanation of the ice ages than the Milankovitch cycles.[121][122]
https://en.wikipedia.org/wiki/Sun
The Gaia Catalogue of Nearby Stars, all within 100 parsecs, contains 331,312 stars and is thought to include at least 92% of the stars of stellar spectral type M9 or "earlier" (i.e. hotter).[158]
Coronal heating problem
The temperature of the photosphere is approximately 6,000 K, whereas the temperature of the corona reaches 1000000–2000000 K.[92] The high temperature of the corona shows that it is heated by something other than direct heat conduction from the photosphere.[94]
It is thought that the energy necessary to heat the corona is provided by turbulent motion in the convection zone below the photosphere, and two main mechanisms have been proposed to explain coronal heating.[92] The first is wave heating, in which sound, gravitational or magnetohydrodynamic waves are produced by turbulence in the convection zone.[92] These waves travel upward and dissipate in the corona, depositing their energy in the ambient matter in the form of heat.[161]The other is magnetic heating, in which magnetic energy is continuously built up by photospheric motion and released through magnetic reconnection in the form of large solar flares and myriad similar but smaller events—nanoflares.[162]
Currently, it is unclear whether waves are an efficient heating mechanism. All waves except Alfvén waves have been found to dissipate or refract before reaching the corona.[163] In addition, Alfvén waves do not easily dissipate in the corona. Current research focus has therefore shifted towards flare heating mechanisms.[92]
https://en.wikipedia.org/wiki/Sun
The Little Ice Age (LIA) was a period of regional cooling that occurred after the Medieval Warm Period.[2] It was not a true ice age of global extent. The term was introduced into scientific literature by François E. Matthes in 1939.[3] The time period has been conventionally defined as extending from the 16th to the 19th centuries,[4][5][6] but some experts prefer an alternative timespan from about 1300[7] to about 1850.[8][9][10]
The NASA Earth Observatory notes three particularly cold intervals: one beginning about 1650, another about 1770, and the last in 1850, all separated by intervals of slight warming.[6]The Intergovernmental Panel on Climate Change Third Assessment Report considered that the timing and areas affected by the Little Ice Age suggested largely independent regional climate changes rather than a globally synchronous increased glaciation. At most, there was modest cooling of the Northern Hemisphere during the period.[11]
Several causes have been proposed: cyclical lows in solar radiation, heightened volcanic activity, changes in the ocean circulation, variations in Earth's orbit and axial tilt (orbital forcing), inherent variability in global climate, and decreases in the human population (for example from the Black Death and the epidemics emerging in the Americas upon European contact[12]).
https://en.wikipedia.org/wiki/Little_Ice_Age
An ice age is a long period of reduction in the temperature of Earth's surface and atmosphere, resulting in the presence or expansion of continental and polar ice sheets and alpine glaciers. Earth's climate alternates between ice ages and greenhouse periods, during which there are no glaciers on the planet. Earth is currently in the Quaternary glaciation.[1] Individual pulses of cold climate within an ice age are termed glacial periods (or, alternatively, glacials, glaciations, glacial stages, stadials, stades, or colloquially, ice ages), and intermittent warm periods within an ice age are called interglacials or interstadials.[2]
In glaciology, ice age implies the presence of extensive ice sheets in both northern and southern hemispheres.[3] By this definition, Earth is currently in an interglacial period—the Holocene. The amount of anthropogenic greenhouse gases emitted into Earth's oceans and atmosphere is predicted to prevent the next glacial period for the next 500,000 years, which otherwise would begin in around 50,000 years, and likely more glacial cycles after.[4][5][6]
https://en.wikipedia.org/wiki/Ice_age
Ulysses (US: /juːˈlɪsiːz/, UK: /ˈjuːlɪ-/) is a decommissioned robotic space probe whose primary mission was to orbit the Sun and study it at all latitudes. It was launched in 1990 and made three "fast latitude scans" of the Sun in 1994/1995, 2000/2001, and 2007/2008. In addition, the probe studied several comets. Ulysses was a joint venture of NASA and the European Space Agency (ESA) under leadership of ESA with participation from Canada's National Research Council.[1] The last day for mission operations on Ulysses was 30 June 2009.[2][3]
To study the Sun at all latitudes, the probe needed to change its orbital inclination and leave the plane of the Solar System. To change the orbital inclination of a spacecraft to about 80° requires a large change in heliocentric velocity, the energy to achieve which far exceeded the capabilities of any launch vehicle. To reach the desired orbit around the Sun, the mission's planners chose a gravity assist maneuver around Jupiter, but this Jupiter encounter meant that Ulysses could not be powered by solar cells. The probe was powered instead by a radioisotope thermoelectric generator(RTG).
The spacecraft was originally named Odysseus, because of its lengthy and indirect trajectory to study the solar poles. It was renamed Ulysses, the Latin translation of "Odysseus", at ESA's request in honor not only of Homer's mythological hero but also of Dante's character in the Inferno.[4] Ulysses was originally scheduled for launch in May 1986 aboard the Space ShuttleChallenger on STS-61-F. Due to the loss of Challenger, the launch of Ulysses was delayed until 6 October 1990 aboard Discovery (mission STS-41).
https://en.wikipedia.org/wiki/Ulysses_(spacecraft)
A phosphene is the phenomenon of seeing light without light actually entering the eye. The word phosphene comes from the Greek words phos (light) and phainein (to show).[1] Phosphenes that are induced by movement or sound may be associated with optic neuritis.[2][3]
Phosphenes can be directly induced by mechanical, electrical, or magnetic stimulation of the retina or visual cortex, or by random firing of cells in the visual system. Phosphenes have also been reported by meditators[4] (called nimitta), people who endure long periods without visual stimulation (the prisoner's cinema), or those who ingest psychedelic drugs.[5]
Phosphenes have been created by electrical stimulation of the brain, reported by neurologist Otfrid Foerster as early as 1929. Brindley and Lewin (1968) inserted a matrix of stimulating electrodes directly into the visual cortex of a 52-year-old blind female, using small pulses of electricity to create phosphenes. These phosphenes were points, spots, and bars of colorless or colored light.[13] Brindley and Rushton (1974) used the phosphenes to create a visual prosthesis, in this case by using the phosphenes to depict Braille spots.
In recent years, researchers have successfully developed experimental brain–computer interfaces or neuroprostheses that stimulate phosphenes to restore vision to people blinded through accidents. Notable successes include the human experiments by William H. Dobelle[14] and Mark Humayun and animal research by Dick Normann.
A noninvasive technique that uses electrodes on the scalp, transcranial magnetic stimulation, has also been shown to produce phosphenes.[15]
Experiments with humans have shown that when the visual cortex is stimulated above the calcarine fissure, phosphenes are produced in the lower part of the visual field, and vice versa.[16]
Phosphenes have been produced by intense, changing magnetic fields, such as with transcranial magnetic stimulation (TMS). These fields can be positioned on different parts of the head to stimulate cells in different parts of the visual system. They also can be induced by alternating currents that entrain neural oscillation as with transcranial alternating current stimulation.[17] In this case they appear in the peripheral visual field.[17] This claim has been disputed. The alternative hypothesis is that current spread from the occipital electrode evokes phosphenes in the retina.[18][19][20] Phosphenes created by magnetic fields are known as magnetophosphenes.
Astronauts exposed to radiation in space have reported seeing phosphenes.[21] Patients undergoing radiotherapy have reported seeing blue flashes of light during treatment; the underlying phenomenon has been shown to resemble Cherenkov radiation.[22]
Phosphenes can be caused by some medications, such as Ivabradine.[23]
Most vision researchers believe that phosphenes result from the normal activity of the visual system after stimulation of one of its parts from some stimulus other than light. For example, Grüsser et al. showed that pressure on the eye results in activation of retinal ganglion cells in a similar way to activation by light.[24] An ancient, discredited theory is that light is generated in the eye.[6] A version of this theory has been revived, except, according to its author, that "phosphene lights are [supposed to be] due to the intrinsic perception of induced or spontaneous increased biophoton emission of cells in various parts of the visual system (from retina to cortex)"[25]
Glutamate; triggering high dose incapacitant and DIW, etc.. upreg gene. G-prot.
https://en.wikipedia.org/wiki/Phosphene
The calcarine sulcus (or calcarine fissure) is an anatomical landmark located at the caudal end of the medial surface of the brain of humans and other primates. Its name comes from the Latin "calcar" meaning "spur".[1] It is very deep and known as a complete sulcus.
https://en.wikipedia.org/wiki/Calcarine_sulcus
Entoptic phenomena (from Ancient Greek ἐντός "within" and ὀπτικός "visual") are visual effects whose source is within the eye itself. (Occasionally, these are called entopic phenomena, which is probably a typographical mistake.)
In Helmholtz's words: "Under suitable conditions light falling on the eye may render visible certain objects within the eye itself. These perceptions are called entoptical."
https://en.wikipedia.org/wiki/Entoptic_phenomenon
No comments:
Post a Comment